Abstract
Background
An increasing body of observational studies have indicated an association between gut microbiota and endometriosis. However, the causal relationship between them is not yet clear. In this study, we employed Mendelian randomization method to investigate the causal relationship between 211 gut microbiota taxa and endometriosis.
Methods
Independent genetic loci significantly associated with the relative abundance of 211 gut microbiota taxa, based on predefined thresholds, were extracted as instrumental variables. The primary analytical approach employed was the IVW method. Effect estimates were assessed primarily using the odds ratio and 95% confidence intervals. Supplementary analyses were conducted using MR-Egger regression, the weighted median method, the simple mode and the weighted mode method to complement the IVW results. In addition, we conducted tests for heterogeneity, horizontal pleiotropy, sensitivity analysis, and MR Steiger to assess the robustness of the results and the strength of the causal relationships.
Results
Based on the IVW method, we found that the family Prevotellaceae, genus Anaerotruncus, genus Olsenella, genus Oscillospira, and order Bacillales were identified as risk factors for endometriosis, while class Melainabacteria and genus Eubacterium ruminantium group were protective factors. Additionally, no causal relationship was observed between endometriosis and gut microbiota. Heterogeneity tests, pleiotropy tests, and leave-one-out sensitivity analyses did not detect any significant heterogeneity or pleiotropic effects.
Conclusions
Our MR study has provided evidence supporting a potential causal relationship between gut microbiota and endometriosis, and it suggests the absence of bidirectional causal effects. These findings could potentially offer new insights for the development of novel strategies for the prevention and treatment of endometriosis.
Similar content being viewed by others
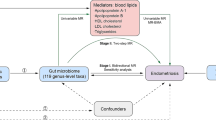
Introduction
Endometriosis (EMs) is a chronic, estrogen-dependent inflammatory condition characterized by the presence of endometrial tissue outside the uterus [1]. Approximately 6–10% of women of reproductive age are affected by EMs, and about 50% of infertile women have EMs [2, 3]. Due to the secretive and diverse nature of EMs symptoms, and the lack of reliable non-invasive methods for detecting endometriosis, it often goes unnoticed. In recent years, the gut microbiota has emerged as a research hotspot, with scholars [4,5,6] discovering its associations with various diseases such as gastrointestinal disorders, cardiovascular diseases, respiratory diseases, and more. Research on the relationship between gut microbiota and endometriosis has spanned over two decades, starting as early as the 1990s and continuing to the present day. Many scholars have observed significant differences in the types, distribution, and abundance of gut microbiota between patients with EMs and healthy women [7, 8]. Additionally, up to 90% of EMs patients experience gastrointestinal issues such as nausea, vomiting, diarrhea, and bloating [9], suggesting a potential imbalance in the gut microbiota. In fact, in a large-scale study, EMs patients were found to have a 50% increased risk of develo** inflammatory bowel disease (IBD) compared to the general population [10]. Furthermore, ecological imbalances in the gut, vagina, or uterus in EMs patients may impact estrogen metabolism, immune system balance, and exacerbate the condition [11, 12]. However, in observational studies, the relationship between gut microbiota and endometriosis can be influenced by confounding factors (such as age and surgical history) and reverse causality, making it uncertain whether these associations are causal in nature.
Randomized controlled trials (RCTs) are considered the gold standard in epidemiology for inferring causal relationships. However, due to ethical constraints, implementing RCTs can be challenging [13]. Mendelian randomization (MR) utilizes single nucleotide polymorphism (SNP) loci as instrumental variables to infer causal associations between exposures and outcomes. It does so by adhering to the genetic principle of “random allocation of parental alleles to offspring,” achieving similar randomization effects without being influenced by external environmental factors, thus compensating for the limitations of observational studies [14].
Currently, there are no MR reports regarding a causal relationship between gut microbiota and endometriosis. Although previous observational studies have suggested an association between gut microbiota and the incidence and progression of endometriosis, the causal relationship is not yet clear. This study is the first application of a two-sample Mendelian randomization approach to explore the causal association between gut microbiota and endometriosis. It aims to provide new insights into the treatment and prevention of endometriosis.
Materials and methods
Research design
In a scenario where the genome wide association study (GWAS) summary data for the exposure variable and the GWAS summary data for the outcome variable are mutually independent, this study employed the TwoSampleMR package in R programming language to conduct a two-sample bidirectional Mendelian randomization analysis. The objective was to investigate the causal association between gut microbiota and endometriosis, with the specific design as shown in Fig. 1. MR analysis adheres to three crucial assumptions [15]: First, the instrumental variables are strongly correlated with the exposure variable. Second, the instrumental variables are independent of observed or unobserved confounding factors. Third, the instrumental variables affect the outcome solely through the exposure.
Data source
The GWAS summary data for endometriosis were obtained from the Finngen database, which includes data from 77,257 European participants and covers 16,377,306 SNPs (https://gwas.mrcieu.ac.uk/datasets/finn-b-N14_ENDOMETRIOSIS/). The statistical data on gut microbiota were derived from the research conducted by the MiBioGen Consortium (http://www.mibiogen.org/), which incorporated 18,340 individuals from 24 cohorts, mainly from Europe [16]. Microbial composition was analyzed using three distinct variable regions of the targeted 16 S rRNA gene, namely V4 (10,413 samples, 13 cohorts), V3-V4 (4,211 samples, 6 cohorts), and V1-V2 (3,716 samples, 5 cohorts). Supplementary File 1 shows a description of the participants in each cohort in a dataset of gut microbiota. Both gut microbiota and endometriosis were selected as exposure and outcome variables, respectively, for the MR analysis. As our study is based on publicly available databases, ethical committee approval was not required.
Instrumental variable selection
(1) IVs Selection: To obtain strongly related exposure data, SNPs with a significance level of P < 5 × 10− 8 were selected as conditions. Given that gut microbiota SNPs rarely have P < 5 × 10− 8, gut microbiota SNPs were selected with a threshold of P < 1 × 10− 5. (2) Independence Criterion: The PLINK aggregation method was used to calculate linkage disequilibrium (LD) between each risk factor’s SNPs. SNPs with an LD coefficient r2 > 0.001 and a physical distance of less than 10,000 kb were removed to ensure that the SNPs were mutually independent and to eliminate the influence of genetic pleiotropy on the results [17, 18]. (3) Statistical Strength Criteria: The strength of the instrumental variables was calculated using the F-statistic, with the formula: F = β2 / SE2 (where β is the allele effect size and SE is the standard error). Instrumental variables with F < 10 were removed to ensure that the instrumental variables were unrelated to unmeasured confounding factors [19]. Finally, the “harmonise_data” function from the TwoSampleMR package was used to align the direction of alleles between exposure and outcome, remove palindromic and incompatible SNPs [20], and exclude SNPs with confounding factors through the PhenoScanner database (http://www.phenoscanner.medschl.cam.ac.uk/).
Mendelian randomization analysis
In this study, the inverse variance weighted (IVW) method [21] was employed as the primary analytical approach for establishing causal relationships. This method, assuming the validity of all instrumental variables, calculates weighted estimates by taking the reciprocal of their variances as weights. It provides the most accurate results when there is no heterogeneity or horizontal pleiotropy present. Additionally, MR-Egger regression, the weighted median (WME) method, the simple mode (SM) and the weighted mode (WM) method were used as supplementary analyses to complement the IVW results. MR-Egger regression method performs weighted linear regression of the exposure and outcome effect estimates, providing a causal effect assessment even when all SNPs are invalid instruments. The WME method leverages the intermediate effects of all available genetic variations, estimating them by weighting each SNP by the inverse variance of its correlation with the outcome. SM and WM are mode-based methods. The mode-based estimation model clusters SNPs with similar causal effects and returns causal effect estimates for the majority of clustered SNPs. Specifically, WM weights the influence of each SNP on the cluster by the inverse variance of its outcome effect. These methods complement the IVW results and provide additional insights into the causal relationships between exposure and outcome variables. Finally, we conducted reverse MR analysis for EMs and gut microbiota. The methods and settings used in these reverse MR analysis were consistent with those of forward MR.
Sensitivity analysis
Heterogeneity testing [22] assesses the presence of differences among various IVs. It utilizes the P-value from Cochran’s Q test to evaluate heterogeneity, with P > 0.05 indicating the absence of heterogeneity. If heterogeneity is detected, the MR pleiotropy residual sum and outlier (MR-PRESSO) test is employed to assess potential outliers [23], eliminate them, and then reanalyze the data. Multiplicity testing [24] verifies the reliability of MR analysis results. MR-Egger intercept is used to detect horizontal pleiotropy, with P > 0.05 indicating the absence of horizontal pleiotropy and, thus, the reliability of the MR analysis results. Sensitivity testing [25] is conducted using a “leave-one-out” approach, sequentially removing each SNP. If the MR results derived from the remaining SNPs do not exhibit significant differences from the overall result, it demonstrates the robustness of the MR results. Furthermore, the MR Steiger directional test was employed to further assess the correlation between the exposure and the outcome.
Results
Causal effect of gut microbiota on EMs
In this study, 211 gut microbiota relative abundances were selected as the exposure variable from gut microbiota GWAS data involving 18,340 participants. These 211 taxa include 9 phylums, 16 classes, 20 orders, 35 families, and 131 genuses. As both heterogeneity and pleiotropy tests yielded negative results, the IVW analysis results were considered the primary reference indicator. The MR analysis results indicate that seven different gut microbiota at various taxonomic levels (1 class, 1 order, 1 family, and 4 genuses) may be associated with endometriosis, as shown in Fig. 2. The main MR analysis results for the association between all gut microbiota and the risk of EMs, as well as the results of heterogeneity and pleiotropy tests, can be found in Supplementary File 2.
We identified associations between endometriosis and five microbial taxonomic groups with positive correlations: family Prevotellaceae (OR = 1.19, 95%CI 1.02 ∼ 1.40, P = 0.026), genus Anaerotruncus (OR = 1.25, 95%CI 1.03 ∼ 1.53, P = 0.025), genus Olsenella (OR = 1.11, 95%CI 1.01 ∼ 1.22, P = 0.036), genus Oscillospira (OR = 1.21, 95%CI 1.01 ∼ 1.46, P = 0.035), order Bacillales (OR = 1.11, 95%CI 1.00 ∼ 1.22, P = 0.042). Simultaneously, two microbial taxonomic groups showed negative associations with endometriosis: class Melainabacteria (OR = 0.86, 95%CI 0.75 ∼ 0.99, P = 0.036), genus Eubacterium ruminantium group (OR = 0.88, 95%CI 0.79 ∼ 0.98, P = 0.015) (Figs. 2, 3 and 4). For detailed results of all SNPs related to these seven gut microbiota (including specific chromosomes, F values, and R2), please refer to Supplementary File 3.
As indicated in Supplementary File 3, we noted that the contribution of total variation (R2 values) for the 7 gut microbiota ranged from 0.13 to 0.21%, with F values spanning from 18.27 to 29.81. This range effectively rules out the possibility of weak genetic instrumental variables. Heterogeneity testing was conducted with a distribution = 10,000 setting. The Cochran’s Q test for both IVW and MR-Egger regressions indicated the absence of heterogeneity among the SNPs of each microbial taxonomic group. Multiple-effect tests revealed that the MR-Egger regression intercepts were all less than 0.05, and their P-values were greater than 0.05, suggesting the absence of horizontal pleiotropy. Furthermore, all MR Steiger directional tests consistently indicated that the direction from gut microbiota to endometriosis was robust for all outcomes (Table 1). Sensitivity analysis was performed using a “leave-one-out” test, and a forest plot was generated. The results indicated that removing any single SNP did not significantly influence the remaining SNP results, all remained on the same side of the null line. This suggests that the MR results in this study are robust. Refer to Fig. 5 for visualization of the sensitivity analysis results.
Reverse-direction MR analyses
Finally, a reverse mendelian randomization analysis was conducted, with endometriosis as the exposure factor and gut microbiota as the outcome variables. The results of each SNP of endometriosis and 7 gut microbiota are shown in Supplementary File 4. Heterogeneity and multiple-effect tests yielded negative results. The IVW analysis revealed that there is no causal relationship between endometriosis and the seven different gut microbiota at various taxonomic levels. The MR Steiger directional tests for the 7 gut microbiota with respect to endometriosis yielded TRUE results. Detailed results can be found in Table 2.
Discussion
Main findings and interpretation
In this study, we assessed for the first time the potential relationship between gut microbiota and endometriosis by a bidirectional MR method, and identified the presence of specific microbial groups at the level of phylum, order, family, and genus that are closely related to EMs, family Prevotellaceae, genus Anaerotruncus, genus Olsenella, genus Oscillospira and order Bacillales had a risk effect on endometriosis, and class Melainabacteria, genus Eubacterium ruminantium group was a protective factor against endometriosis. Sensitivity analyses showed no horizontal pleiotropy, indicating that our MR analyses were not affected by confounding factors, and “leave-one-out” analyses confirmed the robustness of the study.
During menstruation, when endometrial tissue retrogrades into the peritoneal cavity and implants into surrounding tissues, such as the intestines or peritoneum, it leads to the formation of endometriotic lesions [26]. In approximately 10% of women, the immune system fails to clear these ectopic endometrial cells, leading to the activation of macrophages, secretion of pro-inflammatory cytokines and growth factors, and the spread of the lesions [27, 28]. The gut microbiota is a crucial component of the human immune system, with immunomodulatory functions mediated through interactions with stromal cells and epithelial cells. Research has shown that microbial metabolites act as messengers between the gut microbiota and immune functions [29,30,31]. In studies involving mice with endometriosis, alterations in microbial metabolites were observed. The consumption of gut microbiota suppressed inflammation related to endometriosis [32] and influenced immune cell populations, suggesting that gut microbiota can influence endometriosis through immune pathways.
The abnormal endocrine microenvironment within EMs lesions is considered a key characteristic of endometriosis. Estrogen [33] has a direct cell anti-apoptotic and proliferative effect on EMs lesions and promotes the formation of a pro-inflammatory microenvironment, contributing to the chronic progression of the disease. Estrogen is a major regulatory factor for gut microbiota, and the gut microbiome’s genetic repertoire involved in estrogen metabolism is often referred to as the “estrobolome” [34]. It participates in estrogen regulation by secreting beta-glucuronidase [35], forming the estrogen-gut microbiota axis. Research has shown significant differences in the expression of 17β-estradiol, 16-keto-17β-estradiol, 2-hydroxyestrone, and 2-hydroxyestradiol in individuals with EMs. Additionally, there is a clear positive correlation between the gut microbiota of EMs patients and urinary estrogen levels [36]. Family Prevotellaceae belongs to the Bacteroidetes phylum, and a meta-analysis [37] found that the abundance of Bacteroidetes is positively correlated with estrogen levels. When the Firmicutes/Bacteroidetes ratio in the gut decreases, there is an increase in the secretion of beta-glucuronidase in the intestine, leading to elevated estrogen levels. High estrogen levels are directly associated with the development of EMs, and our study provides similar findings.
Multiple studies have indicated [7, 33] that individuals with endometriosis experience dysbiosis in their gut microbiota. The gut microbiota, when fermenting carbohydrates, produces short-chain fatty acids (SCFAs) that can activate G protein-coupled receptors. This activation has beneficial effects by reducing food intake, improving insulin sensitivity, inhibiting fat accumulation, and reducing systemic inflammation [38]. However, in cases of gut microbiota dysbiosis, there is a reduction in SCFA production. Simultaneously, certain neuroactive metabolites, such as glutamate and butyric acid, increase in level. These metabolites can stimulate brain neurons and, through the hypothalamus-pituitary-ovary axis, increase ovarian estrogen secretion, exacerbating the condition of patients [39, 40].
It is noteworthy that PERROTTA et al. [41] established an EM classification model based on random forest, revealing that the vaginal microbiota could predict the severity of endometriomas (EMs), with Anaerococcus identified as the most crucial factor, while the gut microbiota lacked corresponding accuracy. Furthermore, CHEN et al. [42] built a model based on the female reproductive tract microflora, which can distinguish whether infertility is caused by EMs. Considering the potential influences on the gut microbiota from factors such as diet, antimicrobial drugs, and psychological stress, relying on it as a tool for early diagnosis and screening of EMs is unreliable. Similarly, the reproductive tract microbiota can be affected by different physiological stages and diseases like vaginal infections. Therefore, exploration of non-invasive diagnostic methods for EMs is still needed, and using saliva for diagnosis may be more helpful [43]. However, what can be confirmed is the causal association between gut microbiota and endometriosis, with a dynamic interplay between the two, which holds potential implications for future bacteria-based therapies.
Limitation
However, our study has several limitations: (1) Human behavior is complex, and while understanding the genetic risk of a disease can help prevent its occurrence to some extent, environmental factors also play a role in the development of the disease [44], and MR can only partially eliminate the interference of confounding factors such as the environment [45]. (2) The current study may not comprehensively explore the entire spectrum of the gut microbiota, from phylum to genus level, potentially missing other microbial taxa that could have a causal relationship with endometriosis, especially those associated with increased risk. (3) The outcome data used in the study is derived from European populations, and caution should be exercised when extrapolating the results to other populations with different lifestyles, cultural backgrounds, and genetic backgrounds, as specific traits may vary across different racial and ethnic groups driven by their distinct living environments and genetic backgrounds. Efforts should be made to include populations of all ethnicities globally in genetic studies of this nature. (4) Although we have demonstrated a causal relationship between gut microbiota and endometriosis, the underlying mechanism is still unclear and requires further research.
Conclusions
The study collected data from GWAS databases and used a two-sample bidirectional MR approach to confirm the potential causal relationship between gut microbiota and endometriosis, providing new insights into the pathogenesis and treatment of endometriosis. Future research should aim to further elucidate the underlying mechanisms by which these microbial communities influence endometriosis, explore potential treatment strategies targeting gut microbiota.
Data availability
All data generated or analysed during this study are included in this published article and its supplementary information files.
Abbreviations
- MR:
-
Mendelian randomization
- EMs:
-
Endometriosis
- RCT:
-
Randomized controlled trials
- SNPs:
-
Single nucleotide polymorphisms
- IVs:
-
Instrumental variables
- GWAS:
-
Genome wide association study
- LD:
-
Linkage disequilibrium
- IVW:
-
Inverse variance weighted
- WM:
-
Weighted median
References
Filby CE, Rombauts L, Montgomery GW, Giudice LC, Gargett CE. Cellular origins of endometriosis: towards Novel Diagnostics and therapeutics. Semin Reprod Med. 2020;38:201–15.
Engemise S, Gordon C, Konje JC, Endometriosis. BMJ. 2010;340:c2168.
La Rosa VL, Barra F, Chiofalo B, Platania A, Di Guardo F, Conway F, et al. An overview on the relationship between endometriosis and infertility: the impact on sexuality and psychological well-being. J Psychosom Obstet Gynaecol. 2020;41:93–7.
Barandouzi ZA, Starkweather AR, Henderson WA, Gyamfi A, Cong XS. Altered composition of Gut Microbiota in Depression: a systematic review. Front Psychiatry. 2020;11:541.
Marques FZ, Jama HA, Tsyganov K, Gill PA, Rhys-Jones D, Muralitharan RR, et al. Guidelines for transparency on gut Microbiome studies in essential and experimental hypertension. Hypertension. 2019;74:1279–93.
Woodall CA, McGeoch LJ, Hay AD, Hammond A. Respiratory tract infections and gut microbiome modifications: a systematic review. PLoS ONE. 2022;17:e0262057.
Bauşic AIG, Creţoiu SM, Bauşic V, Matasariu DR, Stănculescu RV, Brătilă E. The role of gut dysbiosis in endometriosis’ diagnosis and treatment approaches - case report. Rom J Morphol Embryol. 2023;64:263–9.
Iavarone I, Greco PF, La Verde M, Morlando M, Torella M, de Franciscis P, et al. Correlations between Gut Microbial Composition, Pathophysiological and Surgical aspects in Endometriosis: a review of the literature. Med (Kaunas). 2023;59:347.
Benagiano G, Brosens I, Lippi D. Endometriosis: ancient or modern disease? Indian J Med Res. 2015;141:236–8.
Chiaffarino F, Cipriani S, Ricci E, Roncella E, Mauri PA, Parazzini F, et al. Endometriosis and inflammatory bowel disease: a systematic review of the literature. Eur J Obstet Gynecol Reprod Biol. 2020;252:246–51.
Forbes JD, Chen CY, Knox NC, Marrie RA, El-Gabalawy H, de Kievit T, et al. A comparative study of the gut microbiota in immune-mediated inflammatory diseases-does a common dysbiosis exist? Microbiome. 2018;6:221.
West CE, Renz H, Jenmalm MC, Kozyrskyj AL, Allen KJ, Vuillermin P, et al. The gut microbiota and inflammatory noncommunicable diseases: associations and potentials for gut microbiota therapies. J Allergy Clin Immunol. 2015;135:3–13.
Hariton E, Locascio JJ. Randomised controlled trials - the gold standard for effectiveness research: study design: randomised controlled trials. BJOG. 2018;125:1716.
Carnegie R, Zheng J, Sallis HM, Jones HJ, Wade KH, Evans J, et al. Mendelian randomisation for nutritional psychiatry. Lancet Psychiatry. 2020;7:208–16.
Lawlor DA, Harbord RM, Sterne JA, Timpson N, Davey Smith G. Mendelian randomization: using genes as instruments for making causal inferences in epidemiology. Stat Med. 2008;27:1133–63.
Kurilshikov A, Medina-Gomez C, Bacigalupe R, Radjabzadeh D, Wang J, Demirkan A, et al. Large-scale association analyses identify host factors influencing human gut microbiome composition. Nat Genet. 2021;53:156–65.
Hemani G, Zheng J, Elsworth B, Wade KH, Haberland V, Baird D, et al. The MR-Base platform supports systematic causal inference across the human phenome. Elife. 2018;7:e34408.
Slatkin M. Linkage disequilibrium–understanding the evolutionary past and map** the medical future. Nat Rev Genet. 2008;9:477–85.
Zhuang Z, Yu C, Guo Y, Bian Z, Yang L, Millwood IY, et al. Metabolic Signatures of Genetically Elevated Vitamin D among Chinese: observational and mendelian randomization study. J Clin Endocrinol Metab. 2021;106:e3249–60.
Hartwig FP, Davies NM, Hemani G, Davey Smith G. Two-sample mendelian randomization: avoiding the downsides of a powerful, widely applicable but potentially fallible technique. Int J Epidemiol. 2016;45:1717–26.
Wu F, Huang Y, Hu J, Shao Z. Mendelian randomization study of inflammatory bowel disease and bone mineral density. BMC Med. 2020;18:312.
Bowden J, Spiller W, Del Greco MF, Sheehan N, Thompson J, Minelli C, et al. Improving the visualization, interpretation and analysis of two-sample summary data mendelian randomization via the Radial plot and radial regression. Int J Epidemiol. 2018;47:2100.
Verbanck M, Chen CY, Neale B, Do R. Publisher correction: detection of widespread horizontal pleiotropy in causal relationships inferred from mendelian randomization between complex traits and diseases. Nat Genet. 2018;50:1196.
Carter AR, Sanderson E, Hammerton G, Richmond RC, Davey Smith G, Heron J, et al. Mendelian randomisation for mediation analysis: current methods and challenges for implementation. Eur J Epidemiol. 2021;36:465–78.
Gronau QF, Wagenmakers EJ. Limitations of bayesian leave-one-out Cross-validation for Model Selection. Comput Brain Behav. 2019;2:1–11.
Giudice LC. Clinical practice. Endometriosis. N Engl J Med. 2010;362:2389–98.
Ahn SH, Monsanto SP, Miller C, Singh SS, Thomas R, Tayade C. Pathophysiology and Immune Dysfunction in Endometriosis. Biomed Res Int. 2015;2015:795976.
Han SJ, O’Malley BW. The dynamics of nuclear receptors and nuclear receptor coregulators in the pathogenesis of endometriosis. Hum Reprod Update. 2014;20:467–84.
Arpaia N, Rudensky AY. Microbial metabolites control gut inflammatory responses. Proc Natl Acad Sci U S A. 2014;111:2058–9.
Marsland BJ. Regulating inflammation with microbial metabolites. Nat Med. 2016;22:581–3.
Chang PV, Hao L, Offermanns S, Medzhitov R. The microbial metabolite butyrate regulates intestinal macrophage function via histone deacetylase inhibition. Proc Natl Acad Sci U S A. 2014;111:2247–52.
Chadchan SB, Cheng M, Parnell LA, Yin Y, Schriefer A, Mysorekar IU, et al. Antibiotic therapy with metronidazole reduces endometriosis disease progression in mice: a potential role for gut microbiota. Hum Reprod. 2019;34:1106–16.
Shan J, Ni Z, Cheng W, Zhou L, Zhai D, Sun S, et al. Gut microbiota imbalance and its correlations with hormone and inflammatory factors in patients with stage 3/4 endometriosis. Arch Gynecol Obstet. 2021;304:1363–73.
Qi X, Yun C, Pang Y, Qiao J. The impact of the gut microbiota on the reproductive and metabolic endocrine system. Gut Microbes. 2021;13:1–21.
Ervin SM, Li H, Lim L, Roberts LR, Liang X, Mani S, et al. Gut microbial β-glucuronidases reactivate estrogens as components of the estrobolome that reactivate estrogens. J Biol Chem. 2019;294:18586–99.
Le N, Cregger M, Brown V, Loret de Mola J, Bremer P, Nguyen L, et al. Association of microbial dynamics with urinary estrogens and estrogen metabolites in patients with endometriosis. PLoS ONE. 2021;16:e0261362.
d’Afflitto M, Upadhyaya A, Green A, Peiris M. Association between sex hormone levels and gut microbiota composition and Diversity-A systematic review. J Clin Gastroenterol. 2022;56:384–92.
Cong J, Zhou P, Zhang R. Intestinal microbiota-derived short chain fatty acids in host health and disease. Nutrients. 2022;14:1977.
Mikhael S, Punjala-Patel A, Gavrilova-Jordan L. Hypothalamic-pituitary-ovarian Axis disorders Impacting Female Fertility. Biomedicines. 2019;7:5.
Baj A, Moro E, Bistoletti M, Orlandi V, Crema F, Giaroni C. Glutamatergic signaling along the Microbiota-Gut-Brain Axis. Int J Mol Sci. 2019;20:1482.
Perrotta AR, Borrelli GM, Martins CO, Kallas EG, Sanabani SS, Griffith LG, et al. The vaginal microbiome as a Tool to predict rASRM Stage of Disease in Endometriosis: a pilot study. Reprod Sci. 2020;27:1064–73.
Chen C, Song X, Wei W, Zhong H, Dai J, Lan Z, et al. The microbiota continuum along the female reproductive tract and its relation to uterine-related diseases. Nat Commun. 2017;8:875.
Bendifallah S, Dabi Y, Suisse S, Delbos L, Spiers A, Poilblanc M, et al. Validation of a salivary miRNA signature of endometriosis-interim data. NEJM Evid. 2023;2:EVIDoa2200282.
Meisel SF, Beeken RJ, van Jaarsveld CH, Wardle J. Genetic susceptibility testing and readiness to control weight: results from a randomized controlled trial. Obes (Silver Spring). 2015;23:305–12.
Agustí A, Melén E, DeMeo DL, Breyer-Kohansal R, Faner R. Pathogenesis of chronic obstructive pulmonary disease: understanding the contributions of gene-environment interactions across the lifespan. Lancet Respir Med. 2022;10:512–24.
Acknowledgements
This work benefited from the publicly available statistics of GWAS. We thank the contributors to the original GWAS database.
Funding
This work was supported by the Natural Science Foundation of China (No.82104917), the Natural Science Foundation of Shandong Province (No.ZR2021MH079).
Author information
Authors and Affiliations
Contributions
Pengfei Liu and **xing Liu conceived the study. Chunxiao Dang and Yuyan Chai provided the design of the study. Zhenting Chen and Pengfei Liu collected the data. **ao Yu and Yan Liu conducted the main analyses of the study. Chunxiao Dang and Zhenting Chen wrote the body of the manuscript. Yan Liu and **xing Liu revised the manuscript. All authors reviewed the the manuscript.
Corresponding authors
Ethics declarations
Ethics approval and consent to participate
Not applicable.
Consent for publication
Not applicable.
Competing interests
The authors declare no competing interests.
Additional information
Publisher’s Note
Springer Nature remains neutral with regard to jurisdictional claims in published maps and institutional affiliations.
Electronic supplementary material
Below is the link to the electronic supplementary material.
Rights and permissions
Open Access This article is licensed under a Creative Commons Attribution 4.0 International License, which permits use, sharing, adaptation, distribution and reproduction in any medium or format, as long as you give appropriate credit to the original author(s) and the source, provide a link to the Creative Commons licence, and indicate if changes were made. The images or other third party material in this article are included in the article’s Creative Commons licence, unless indicated otherwise in a credit line to the material. If material is not included in the article’s Creative Commons licence and your intended use is not permitted by statutory regulation or exceeds the permitted use, you will need to obtain permission directly from the copyright holder. To view a copy of this licence, visit http://creativecommons.org/licenses/by/4.0/. The Creative Commons Public Domain Dedication waiver (http://creativecommons.org/publicdomain/zero/1.0/) applies to the data made available in this article, unless otherwise stated in a credit line to the data.
About this article
Cite this article
Dang, C., Chen, Z., Chai, Y. et al. Assessing the relationship between gut microbiota and endometriosis: a bidirectional two-sample mendelian randomization analysis. BMC Women's Health 24, 123 (2024). https://doi.org/10.1186/s12905-024-02945-z
Received:
Accepted:
Published:
DOI: https://doi.org/10.1186/s12905-024-02945-z