Abstract
One of the most important parameters of a crude oil recovery process is the interaction of rock-crude oil. In order to alter the positive interaction rock-crude oil, several chemical systems have been proposed. In this study, we use graphene oxide (GO) to alter the positive interaction between sandstone rock and crude oil. In order to evaluate the alteration of the interaction rock oil, we measured the change in the contact angle water sandstone and oil sandstone. In addition, we have measured the interfacial tension (IFT) modification due to the presence of GO in the fluids. The combined effects of graphene oxide concentration, salinity, and pH on interfacial tension and contact angle have been evaluated. The results show that despite GO has not produced significant changes in interfacial tension, the adsorption of GO on the sandstone surface changes the wettability of the sandstone from being strongly crude oil-wet to intermediate crude oil-wettability. Measurements of the contact angle of the treated rock surface with GO solution under soft salinity conditions and pH of 8 show that adsorbed GO can alter the wettability of sandstone from 150 to 90°. In order to corroborate the change in wettability, Amott-Harvey tests were performed. The formation brine and crude oil were subjected to fluid–fluid compatibility tests. These evaluations confirmed a favorable compatibility with the formulation containing 900 ppm of total dissolved solids (TDS), pH 8, and 900 ppm of GO. As a result, it is considered a potential candidate for coreflooding tests.
Similar content being viewed by others
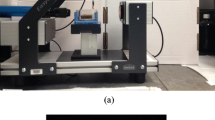
Avoid common mistakes on your manuscript.
1 Introduction
Nanotechnology has opened new frontiers in the realm of enhanced oil recovery (EOR). This innovative approach leverages the properties of nanoparticles to revolutionize the way we extract hydrocarbons from reservoirs. In EOR, nanoparticles are employed to enhance fluid-rock interactions, modify wettability, reduce interfacial tension, and improve sweep efficiency. Nanofluids are defined by the presence of nanoparticles, these nanoparticles exhibit the remarkable ability to disperse uniformly within a liquid medium, such as distilled water and/or brine [1,2,3,4,5,6,7]. The application of enhanced oil recovery (EOR) techniques in sandstone reservoirs holds a paramount position in the oil and gas industry, given that nearly a quarter of the sedimentary rocks of the world are sandstones. Additionally, sandstone presents positive interaction with crude oils; this rock is crude oil-wet [8]. Among the array of techniques available, chemical enhanced oil recovery (CEOR) methods emerge as the predominant choice for tackling the intricacies of crude oil retrieval in such reservoirs [9,10,11,12].
The chemical used in EOR (CEOR) are intended to reduce crude oil/water interfacial tension (IFT), to increase capillary number and altering the wettability [13,14,15,16].
In this context, a theory has been postulated regarding the displacement of crude oil from a solid surface through the dispersion of nanoparticles [12]. Wasan and Nikolov [12] determined that nanoparticles have the potential to generate a wedge-like coating at the point of convergence where the crude oil droplet, nanofluid, and rock surface intersect. This is accomplished by exerting a pressure referred to as structural disjunction force on the peak of the wedge-shaped layer and the interface between the phases. This ultimately separates the crude oil droplet from the rock pore surface and expels it due to capillary force [17, 18].
The interplay between nanoparticles and the subsurface geological formation is a critical factor in enhancing the effectiveness of nanofluids in enhanced oil recovery (EOR) endeavors. A comprehensive comprehension of this element is instrumental in sha** a prosperous EOR initiative aided by nanoparticles. Carefully crafted nanoparticles can be adsorbed at crude oil–water interfaces and on surface of the rock pores and alter the interactions between crude oil and water, rock and water, and crude oil and rock. This alteration of interactions seeks to decrease the IFT of crude oil/water and to increase the wettability of the rock towards the water [19].
In this context, the utilization of amphiphilic materials based on carbon structures in the process of enhanced oil recovery (EOR) has been explored in several research works. Bera and Belhaj [20] explored the implementation of nanotechnology in the upstream petroleum sector as an illustrative case. Through an analysis of laboratory investigations focused on potential applications of nanotechnology in the oilfield, they determined that silica nanoparticles could enhance oil recovery by an extra 10% in coreflooding experiments. Furthermore, in a comparative analysis, Bera [21] assessed the effectiveness of crude oil retrieval using silica nanofluid in the presence of guar gum across a temperature range from 25 to 75 °C. The findings demonstrated that the nanofluid significantly enhanced oil recovery by 44.3% original oil in place (OOIP) [21].
Luo et al. [22, 23] managed to enhance the efficiency of oil recovery in 7.5% using graphene-based amphiphilic at a concentration of 0.005 wt%. They were able to reduce the contact angle from 150 to 79°, changing the rock surface from crude oil-wet to intermediate wettability. Its finding show that the nanofluid proved highly efficient at extremely low concentrations. They also reported the presence of resilient interfacial layers at the interfaces of crude oil and water, which could potentially account for the elevated efficiency in oil recovery. The coreflooding measurement showed displacement efficiency of 69.6% after brine flooding and 15.2% with nanofluid flooding.
In 2017, Li and colleagues [24] introduced a novel nanofluid using luminescent carbon nanoparticles designed for enhanced oil recovery (EOR). This nanofluid demonstrated exceptional proficiency in transforming the wettability of rock surfaces and facilitating the displacement of crude oil through a permeable medium. Within this investigation, the creation and modification of graphene oxide (GO) were carried out to transition the rock’s wettability from being oil-attracting to water-attracting. While prior research has explored the impact of GO nanoparticles on altering wettability in carbonate reservoirs, its comprehensive examination in sandstone reservoirs remains limited. In this study, we use graphene oxide (GO) at different salinities and pH to alter the wettability of sandstones from crude oil-wettable to water-wettable. Also, we show a decrement of IFT due to interfacial properties of GO [25].
2 Experimental section
2.1 Materials
Graphite powder, sodium nitrate, potassium permanganate, 98% sulfuric acid, hydrochloric acid, 30% hydrogen peroxide, sodium chloride (99.5%), sodium hydroxide, and toluene were of analytical grade and were purchased from Merck. The crude oil samples and sandstone cores were obtained from an oil field located in the Colombian northeast zone. The formation brine composition, SARA (Saturated, Aromatics, Resins, Asphaltenes), and API properties of crude oil sample are presented in Table 1.
3 Synthesis of GO
Graphene oxide nanosheets were produced through an adaptation of the Tour-modified Hummer’s method [11]. In summary, 2 g of unadulterated graphite powder were blended in a container along with 120 mL of sulfuric acid. This mixture was continually agitated at 60 °C. Subsequently, 12 g of potassium permanganate were incrementally introduced at a rate of 0.5 g/min, while maintaining the temperature at 60 °C. These conditions persisted for a period of 24 h. Following this, 30% hydrogen peroxide was cautiously added in a drop-wise manner to terminate the oxidation process. Then, 500 mL of deionized water (DW) was incorporated, and the dispersion was allowed to settle at ambient temperature for 8 h without any stirring. Any remaining acids and oxidizing agents within the GO nanosheets were removed through multiple rinses and centrifugation at 8000 rpm for 20 min, adjusted until achieving a pH of 5. The acquired graphene oxide was combined with 500 mL of deionized water (DW) and subsequently underwent exfoliation via ultrasound for a duration of 2 h to produce a GO aqueous dispersion. Finally, the GO dispersion was subjected to freeze-drying at − 30 °C for a 5-day period, followed by lyophilization to obtain the GO powder [2].
4 Preparation of nanofluids
The nanofluids were prepared at three concentrations (300 ppm, 600 ppm, and 900 ppm), salinities (300 ppm, 600 ppm, and 900 ppm), and pH (4, 6, and 8). The stability of the dispersions was evaluated by means of zeta potential measurements and aging tests.
4.1 Characterization of the rock
Sandstone cores obtained from a crude oil field located in the Colombian northeast zone were cleaned with toluene and methanol in a Soxhlet system and then analyzed by scanning electron microscopy (SEM) and energy dispersive spectroscopy (EDS) to know the morphology of sandstone and its elemental composition. As expected, the sandstone rock contains mainly silicon and oxygen (SiO2) (Fig. 1a, b).
4.2 Interfacial tension (IFT) and contact angle measurements
IFT measurements were carried out using a camera of 752 × 582 pixels by the pendant drop method. The pendant drop is one of the most known methods to measure the IFT. Here, a U-shaped needle is required to inject the crude oil droplet at its tip. For this, a cell filled with the nanofluid exposed to a high-intensity light is filled in contrast to a camera; once the crude oil droplet is injected into the nanofluid medium, the image of the droplet shape is recorded using the camera. The image of the droplet is then analyzed and the IFT is quantified.
Before conducting the contact angle measurements, it is essential to carry out a rock wettability restoration protocol. To achieve this, the following steps are performed; the sandstone plugs were cut and cleaned. Then, sandstone plugs were fully saturated with formation brine and aging for 7 days, followed by crude oil saturation and aging for 20 days at 48 °C and atmospheric pressure. The oil-wet substrates were aged for approximately 3 days in the nanofluids to be evaluated (at the different GO concentrations, pH, and salinity). Contact angle measurements were used to determine the wettability of the sandstone rocks.
4.3 Amott-Harvey evaluation
The Amott-Harvey method combines imbibition and forced displacements to measure wettability, based on the fact that the wetting fluid will spontaneously imbibe into the core, displacing the non-wetting fluid. According to the Amott indices, the rock can exhibit either uniform or mixed wettability. Uniform wettability occurs when the rock has a preference for a single fluid or none of the fluids in particular. Mixed wettability occurs when the rock is wetted by both fluids. The Amott-Harvey index establishes ranges to classify the overall wettability of the sample, indicating its preference for water, oil, or neutrality, while also accounting for the effects of mixed or uniform wettability. In Eq. (1), the calculation of the Amott-Harvey index can be observed.
where.
I = Amott-Harvey index;
Iw = Amott index for water;
Io = Amott index for oil;
Vwesp = Volume of water spontaneously displaced by oil imbibition;
Vwfor = Volume of water forcibly displaced by oil injection;
Voesp = Volume of oil spontaneously displaced by water imbibition;
Vofor = Volume of oil forcibly displaced by water injection.
An Amott-Harvey index between 0.3 and 1 indicates a preference for water, an index between − 0.3 and 0.3 indicates neutral wettability, and an index between − 1 and − 0.3 indicates oil-wet wettability.
For this work, a modification of the original method is employed, utilizing a coreflooding setup instead of a centrifuge to perform forced displacements. This modified approach is used for both the brine or graphene oxide formulations displacement stage and the mineral oil displacement stage. The coreflooding equipment enables the simulation of reservoir conditions, such as overburden pressure and temperature. Additionally, it allows for the calculation of the absolute and effective permeability of each injected phase based on Darcy’s law. The schematic representation of the test is shown in the Fig. 2.
4.4 Compatibility tests
Testing the fluid–fluid compatibility of graphene oxide nanofluid with reservoir fluids is a crucial initial step towards its potential use as an enhanced oil recovery method. Compatibility tests allow us to assess the likelihood of nanoparticle agglomeration, changes in fluid properties, and potential formation of emulsions or sludge. Such information is critical in determining the viability of a nanofluid as a displacement agent and helps to prevent operational issues that could negatively affect field performance. Therefore, conducting fluid–fluid compatibility tests is an essential aspect of the development and evaluation of nanofluid-based enhanced oil recovery methods.
4.4.1 Detergence
The “detergency” of sandstone refers to its capacity to release and retain hydrocarbons, such as crude oil, in its porous structure. In other words, detergency is the ability of the rock to separate and release oil from its pores and facilitate its flow towards production wells.
The present study describes a testing procedure to evaluate the detergent capacity of a graphene oxide sample on formation sand in the presence of crude oil and formation water. The test involves adding 5 mL of 40/60 mesh formation sand to a 100 mL transparent Schott glass bottle, along with 50 mL of the graphene oxide sample. The system is left to soak for one hour at room temperature, after which the solution is decanted and crude oil and formation brine are added in a 50:50 ratio. The mixture is gently stirred for 1 min and then brought to reservoir temperature for 1 h. A photographic record is taken and annotations made at the end of the hour.
4.4.2 Sludge Tests
The sludge test determines the tendency of crude oil to form solid precipitates when in contact with generally acidic substances, independent of the tendency to form emulsions. A 50:50 mixture of the sample to be evaluated and clean crude oil (free of solids and emulsion) is placed in a blue cap flask and vigorously agitated for 60 s. The mixture is then held at the formation temperature, if applicable (90 °C or as directed by the Technical Director) for 24 h. In this case, the test was performed at 90 °C. After 24 h, the mixture is passed through a clean 100 mesh sieve, and the presence of solids retained on the sieve is observed. If no solids are observed on the sieve, no sludge has formed. If solids are observed, they are alternately washed with warm water and soltrol 130 (mineral oil). This will remove emulsions and paraffins, but not the sludge caused by the acid. The amount of sludge is described, and photographic records are taken.
5 Results
Thirty interfacial tension (IFT) and contact angle measurements were performed by varying pH, salinity, and GO concentration. A significant 50% reduction in interfacial tension and a shift in rock wettability from approximately 150° to 90° were observed. The formulations that yielded the most favorable results in altering wettability under intermediate conditions are presented in the Table 2.
Based on the best levels from both analysis and experiments, the suitable conditions for decreasing interfacial tension and contact angle are determined to be an initial GO concentration of 900 ppm, a salinity of 900 ppm, and a pH value of 8.
The observed 50% reduction in interfacial tension and the change in rock wettability have several practical implications for industrial applications in enhanced oil recovery (EOR) like improved oil recovery efficiency, reduced interfacial tension between the injected fluid and the reservoir rock can facilitate the displacement of trapped oil, making it more accessible for extraction. This leads to increased oil recovery rates, which are crucial for optimizing production in mature reservoirs.
5.1 GO surface charge and sandstone
When graphene oxide is immersed in water, the carboxylic acid groups on the graphene oxide sheets are ionized. The negative charge on the surface of graphene oxide pertains due to the ionization of these chemical entities. As the pH increases, the negative sites derived from the carboxylic and phenolic groups increase. As a consequence, the surface charge of GO tends to become increasingly negative in alkaline pH conditions; however, increasing salinity from 300 to 900 ppm may neutralize the negative charge suppressing the electric double layer and promoting the GO precipitation.
As observed in the rock analysis, the sandstone used in this study is composed of quartz mineral as the main component. When quartz is immersed in water, Water molecules form hydrogen bonds with silanol (–SiOH) groups on the silica surface, leading to the formation of silanol groups. The formation of –SiO– sites generates negative charges on the quartz surface. Furthermore, the presence of OH groups in GO enables its affinity for silanol groups, but it is important to note that quartz not only possesses silanol groups but also has a crude-oil-affine face composed of siloxanes. Additionally, the surface of GO sheets indicates an abundance of oxygen-containing groups. As a result, both the –OH groups of sandstone and the O-containing groups of GO could undergo ionization at high pH values. Moreover, there may be a possibility of n–π interaction between the –O– groups of sandstone and the π electrons of GO. The presence of electrolytes at high solution pH values enhances the adsorption of GO on sandstone.
5.2 Alteration of the rock surface after GO adsorption
Figure 3 shows the SEM and EDS image of the sandstone before treatment and after the optimal treatment (GO concentration 900 ppm, TDS 900 ppm, and pH 8); it can be observed the GO leaves adsorbed on the surface of the sandstone, as well as in the EDS analysis the presence of GO.
Contact angle measurements were repeated at the best conditions to study the effect of adsorbed GO on altering the wettability of the sandstone surface. As shown in Fig. 4a, the contact angle for the crude oil-wet sandstone rock prior to fluid treatment is approximately 145°, corresponding to a strongly oil-wet condition. Figure 4b shows a decrease in the contact angle due to the treatment with the optimal GO solution, changing the contact angle to approximately 92°, which means that the wettability of the sandstone slices changes from strongly oil-wet to intermediate oil-wet.
5.3 Amott-Harvey Test
The sample is initially immersed in formation water for a specified period (21 days), and the volume of crude oil spontaneously displaced by water imbibition is measured. Subsequently, water is injected until reaching residual oil saturation (\({S}_{or})\), and the volume of crude oil forcibly displaced is measured. The core is then immersed in crude oil for a specified period (21 days), and the volume of formation water spontaneously displaced by crude oil imbibition is measured. Finally, crude oil is injected until reaching irreducible water saturation (\({S}_{wir})\), and the volume of formation water forcibly displaced is measured. In the Fig. 5, the restoration of wettability can be observed.
After calculating the rock wettability, an evaluation of wettability changes due to contact with graphene oxide under optimal conditions (GO 900 ppm, pH 8, and TDS 900 ppm) was conducted, and the results are presented in Table 3.
According to the obtained results, it was confirmed that the rock transitions from an oil-wet condition to a neutral wettability condition after being in contact with the graphene oxide formulation. That is due further fluid–fluid compatibility tests are conducted.
5.4 Compatibility test
The compatibility test allows determining the presence of solids or crystals in the interface and the aqueous phase, by placing the sample to be evaluated in contact with crude oil at reservoir temperature.
5.4.1 Detergence
A control test was conducted using formation sand, crude oil, and brine. The results obtained demonstrate that graphene oxide significantly enhances the detergent capacity, as can be observed in Fig. 6, where the sand treated with graphene oxide is free of crude oil residue in comparison to the control.
5.4.2 Sludge test
The presence of graphene oxide does not cause the formation of sludge upon contact with the crude oil for 24 h at formation temperature (Fig. 7).
6 Conclusions
Due to the adsorption of graphene oxide (GO), the wettability of the rock is altered, changing from very wet conditions due to oil (150°) to intermediate conditions (90°) to the optimum conditions (900 ppm of GO, 900 ppm of TDS, and 8 pH). The practical implications of these findings in industrial applications are increased oil recovery, improved sweep efficiency, potential cost savings, and environmental benefits, all of which can significantly impact the oil and gas industry’s economic and environmental performance.
It was found that at salinities higher than 900 ppm the graphene oxide dispersions were not stable, which is why concentrations below this range were used.
The change in rock wettability upon contact with the 900 ppm GO formulation, at pH 8 and 900 ppm salinity, was confirmed through Amott-Harvey tests. The transition from oil-wet conditions to intermediate conditions, as indicated by the Amott-Harvey index, aligns consistently with the contact angle results.
The presence of OH groups in GO enhances its interaction with silanol groups, highlighting its potential as an effective agent for modifying surface properties. However, it is crucial to recognize that quartz possesses not only silanol groups but also a crude-oil-affine face consisting of siloxanes. The abundance of oxygen-containing groups on the surface of GO sheets suggests the likelihood of ionization for both the –OH groups of sandstone and the O-containing groups of GO at high pH values. Additionally, the possibility of n–π interactions between the –O– groups of sandstone and the π electrons of GO provides further insights into the complex interfacial behavior. These findings open avenues for exploring novel applications and strategies in wettability alteration and surface engineering for various industrial processes.
The results of this study indicate that graphene oxide has great potential as a detergent improver in oil production. A significant improvement in the cleaning capacity of the sand in the presence of crude oil was observed, which could translate into higher oil production. Additionally, it was observed that graphene oxide does not induce sludge formation and does not affect crude oil stability, suggesting good compatibility between the two. Further studies are recommended to evaluate the technical and economic feasibility of graphene oxide application in the oil industry. Overall, these results indicate that the use of nanomaterials such as graphene oxide could have a significant impact on the efficiency and profitability of oil production.
Based on the favorable results obtained from fluid–fluid compatibility tests, the formulation is deemed a suitable candidate for coreflooding experiments.
Data availability
The data that support the findings of this study are available from the corresponding author reasonable request.
References
M.H.U. Bhuiyan, R. Saidur, M.A. Amalina, R.M. Mostafizur, A. Islam, Effect of nanoparticles concentration and their sizes on surface tension of nanofluids. Procedia Engineering 105, 431e437 (2015). https://doi.org/10.1016/j.proeng.2015.05.030
B.A. Arenas-Blanco, E.M. Pérez-Rodríguez, R. Cabanzo Hernández, N. Santos-Santos, E. Mejía-Ospino, Sulfonated graphene oxide nanofluid: potential applications for enhanced oil recovery. Energy & Fuels 35(24), 20071–20078 (2021). https://doi.org/10.1021/acs.energyfuels.1c03329
S.K. Das, S.U.S. Choi, W. Yu, T. Pradeep, Nanofluids: science and technology. John Wiley Sons Inc, Hoboken, New Jersey. (2007). https://doi.org/10.1002/9780470180693
S. Das, A. Katiyar, N. Rohilla, R.T. Bonnecaze, Q.A. Nguyen, Methodology for chemical formulation for wettability alteration induced water imbibition in carbonate reservoirs. J. Petrol. Sci. Eng. 198, 108136 (2021). https://doi.org/10.1016/j.petrol.2020.108136
X. Sun, Y. Zhang, G. Chen, Z. Gai, Application of nanoparticles in enhanced oil recovery: a critical review of recent progress. Energies 10, 1e33 (2017). https://doi.org/10.3390/en10030345
R. Taylor, S. Coulombe, T. Otanicar, P. Phelan, A. Gunawan, W. Lv, G. Rosengarten, R. Prasher, H. Tyagi, Small particles, big impacts: a review of the diverse applications of nanofluids. J. Appl. Phys. 113(1), 11e301 (2013). https://doi.org/10.1063/1.4754271
L. Qi, C. Song, T. Wang, Q. Li, G.J. Hirasaki, R. Verduzco, Polymer-coated nanoparticles for reversible emulsification and recovery of heavy oil. Langmuir 34, 6522e6528 (2018). https://doi.org/10.1021/acs.langmuir.8b00655
Lucas Rego Barros Rebello, João Victor Nicolini, Helen C. Ferraz, Nanofluid formulation based on the combined effect of silica nanoparticles and low salinity water for enhanced oil recovery in sandstones, Geoenergy Science and Engineering, 223 (2023). https://doi.org/10.1016/j.geoen.2023.211570.
A. Alizadeh-Pahlavan, L. Cueto-Felgueroso, A.E. Hosoi, G.H. McKinley, R. Juanes, Thin films in partial wetting: stability, dewetting and coarsening. J Fluid Mech 845, 642e681 (2018). https://doi.org/10.1017/jfm.2018.255
J. Giraldo, P. Benjumea, S. Lopera, F. Cortes, M. Ruiz, Wettability alteration of sandstone cores by alumina-based nanofluids. Energy & Fuels 27, 3659e3665 (2013). https://doi.org/10.1021/ef4002956
R. Kumar, T. Sharma, Stability and rheological properties of nanofluids stabilized by SiO2 nanoparticles and SiO2-TiO2 nanocomposites for oilfield applications. Colloids. Surf. A Physicochem. Eng. Asp. 539, 171e183 (2018). https://doi.org/10.1016/j.colsurfa.2017.12.028
D. Wasan, A.D. Nikolov, Spreading of nanofluids on solids. Nature 423, 156e159 (2003). https://doi.org/10.1038/nature01591
J.A. Ali, K. Kolo, A. Khaksar-Manshad, A.H. Mohammadi, Recent advances in the application of nanotechnology in chemical enhanced oil recovery: effects of nanoparticles on wettability alteration, interfacial tension reduction, and flooding. Egypt. J. Pet. 27(4), 1371–1383 (2018). https://doi.org/10.1016/j.ejpe.2018.09.006
P.A. Khoshkar, M. Fatemi, M.H. Ghazanfari, Static and dynamic evaluation of the effect of nanomaterials on the performance of a novel synthesized PPG for water shut-off and improved oil recovery in fractured reservoirs. J. Petrol. Sci. Eng. 189, 107–19 (2020). https://doi.org/10.1016/j.petrol.2020.107019
F. Sagala, T. Montoya, A. Hethnawi, G. Vitale, N.N. Nassar, Nanopyroxene-based nanofuids for enhanced oil recovery in sandstone cores at reservoir temperature. Energy Fuels 33(2), 877–890 (2019). https://doi.org/10.1021/acs.energyfuels.8b03749
C. Yang, K.C. Leong, Wettability alteration in carbonate reservoirs by carbon nanofluids. Colloids Surf., A (2020). https://doi.org/10.1016/j.colsurfa.2020.124819
B. Peng, L. Zhang, J. Luo, P. Wang, B. Ding, M. Zeng, Z. Cheng, A review of nanomaterials for nanofluid enhanced oil recovery. RSC Adv. 7(51), 32246–32254 (2017). https://doi.org/10.1039/C7RA05592G
H. Radnia, A.M. Rashidi, A.R. Solaimany-Nazar, M.M. Eskandari, M. Jalilian, A novel nano-fluid based on sulfonated graphene for enhanced oil recovery. J. Mol. Liq. 271, 795e806 (2018). https://doi.org/10.1016/j.molliq.2018.09.070
Maraim Kh. Uoda, Hussein Q. Hussein, Rana R. Jalil, Experimental investigation of combined carbon nanoparticles (CNPs), ionic liquid (I.L), and low salinity water to enhance oil recovery (EOR) at Iraq’s southern oil fields, Journal of Molecular Liquids, Volume 391, Part A, 2023, https://doi.org/10.1016/j.molliq.2023.123322.
A. Bera, H. Belhaj, Application of nanotechnology by means of nanoparticles and nanodispersions in oil recovery-a comprehensive review. J. Nat. Gas Sci. Eng. 34, 1284–1309 (2016). https://doi.org/10.1016/j.jngse.2016.08.023
A. Bera, S. Shah, M. Shah, J. Agarwal, R.K. Vij, Mechanistic study on silica nanoparticles-assisted guar gum polymer flooding for enhanced oil recovery in sandstone reservoirs. Colloid. Surface. Physicochem. Eng. Aspect. 598, 124–833 (2020). https://doi.org/10.1016/j.colsurfa.2020.124833
D. Luo, F. Wang, J. Zhu, F. Cao, Y. Liu, X. Li, R.C. Willson, Z. Yang, C.W. Chu, Z. Ren, Nanofluid of graphene- based amphiphilic Janus nanosheets for tertiary or enhanced oil recovery: high performance at low concentration. Proc. Natl. Acad. Sci. Unit. States Am. 113, 7711–7716 (2016). https://doi.org/10.1073/pnas.1608135113
D. Luo, F. Wang, J. Zhu, L. Tang, Z. Zhu, J. Bao, R.C. Willson, Z. Yang, Z. Ren, Secondary oil recovery using graphene-based amphiphilic Janus nanosheet fluid at an ultralow concentration. Ind. Eng. Chem. Res. 56(39), 11125–11132 (2017). https://doi.org/10.1021/acs.iecr.7b02384
Li, Y., Dai, C., Zhou, H., Wang, X., Lv, W., Wu, Y., Zhao, M., 2017. A novel nanofluid based on fluorescent carbon nanoparticles for enhanced oil recovery. Ind. Eng. Chem. Res. 12464–12470. https://doi.org/10.1021/acs.iecr.7b03617.
Stanley Sim Sze Lim, Henry Elochukwu, Jobrun Nandong, Ziad Bennour, Mohamed Ali Hamid, A review on the mechanisms of low salinity water/surfactant/nanoparticles and the potential synergistic application for c-EOR, Petroleum Research, Volume 8, Issue 3, 2023, Pages 324–337, ISSN 2096–2495, https://doi.org/10.1016/j.ptlrs.2023.02.001.
A. Bera, T. Kumar, K. Ojha, A. Mandal, Adsorption of surfactants on sand surface in enhanced oil recovery: isotherms, kinetics and thermodynamic studies. Appl. Surf. Sci. 284, 87–99 (2013). https://doi.org/10.1016/j.apsusc.2013.07.029
D.C. Marcano, D.V. Kosynkin, J.M. Berlin, A. Sinitskii, Z. Sun, A. Slesarev, L.B. Alemany, W. Lu, J.M. Tour, Improved synthesis of graphene oxide. ACS Nano 4(8), 4806–4814 (2010). https://doi.org/10.1021/nn1006368
J. Ji, G. Zhang, H. Chen, S. Wang, G. Zhang, F. Zhang, X. Fan, Sulfonated graphene as water-tolerant solid acid catalyst. Chem. Sci. 2, 484–487 (2011). https://doi.org/10.1039/C0SC00484G
C. Peng, Y. **ong, Z. Liu, F. Zhang, E. Ou, J. Qian, Y. **ong, W. Xu, Bulk functionalization of graphene using diazonium compounds and amide reaction. Appl. Surf. Sci. 280, 914e919 (2013). https://doi.org/10.1016/j.apsusc.2013.05.094
H. Radnia, A.R. Solaimany-Nazar, A.M. Rashidi, Experimental assessment of graphene oxide adsorption onto sandstone reservoir rocks through response surface methodology. J. Taivan. Inst. Chem. E. 80, 34e45 (2017). https://doi.org/10.1016/j.jtice.2017.07.033
A. Rezaei-Namin, A. Rashidi, A.A. Gharesheikhlou, E. Ghasemy, M. Jalilian, Experimental application of functionalized N-doped graphene for improving enhanced oil recovery. Colloid. Surface. Physicochem. Eng. Aspect. 581, 123–801 (2019). https://doi.org/10.1016/j.colsurfa.2019.123801
Acknowledgements
The authors are grateful to the Central Laboratories of the Technological Park of Guatiguará of the Universidad Industrial Santander; Surface Laboratory, Spectroscopy Laboratory, Microscopy Laboratory, and Catalysis Research Center (CICAT) for their assistance.
Funding
Open Access funding provided by Colombia Consortium. This work was supported by Colombian Ministry of Science and Technology (MINCIENCIAS) within the framework of project No. 80740–186-2021.
Author information
Authors and Affiliations
Contributions
All authors contributed to the study conception and design. Material preparation, data collection, and analysis were performed by JLGD, JJRM, NSS, JCPA, and EMO. The first draft of the manuscript was written by JLGD and all authors commented on previous versions of the manuscript. All authors read and approved the final manuscript.
Corresponding author
Rights and permissions
Open Access This article is licensed under a Creative Commons Attribution 4.0 International License, which permits use, sharing, adaptation, distribution and reproduction in any medium or format, as long as you give appropriate credit to the original author(s) and the source, provide a link to the Creative Commons licence, and indicate if changes were made. The images or other third party material in this article are included in the article's Creative Commons licence, unless indicated otherwise in a credit line to the material. If material is not included in the article's Creative Commons licence and your intended use is not permitted by statutory regulation or exceeds the permitted use, you will need to obtain permission directly from the copyright holder. To view a copy of this licence, visit http://creativecommons.org/licenses/by/4.0/.
About this article
Cite this article
Gómez-Delgado, J.L., Rodriguez-Molina, J.J., Perez-Angulo, J.C. et al. Evaluation of the wettability alteration on sandstone rock by graphene oxide adsorption. emergent mater. (2023). https://doi.org/10.1007/s42247-023-00596-8
Received:
Accepted:
Published:
DOI: https://doi.org/10.1007/s42247-023-00596-8