Abstract
The working fluid is a critical component in direct absorption solar collectors. Nanoparticle (NP) suspensions can be used as efficient solar absorption media. In this work, Cu@C core–shell NPs were designed to enhance the solar absorption performance by the coupled effect of the local surface plasmon resonance of the Cu core and the strong intrinsic absorption of the C shell. Results showed that the core radius (rc) and shell thickness (ts) had significant effects on the optical properties of Cu@C NPs. The largest absorption power per unit volume of single Cu@C NP was achieved at rc − ts = 25–4 nm. Taking into account the multi-scattering effect in the NP suspensions, the maximum solar absorption efficiency of Cu@C NP suspensions was obtained as 95.6% at rc − ts = 25–14 nm when the volume fraction fv = 100 ppm and height h = 3 mm. These results indicate that the scattering effect is significant to evaluate the solar absorption performance of NP suspensions and the core–shell size parameters should be considered cautiously for the solar thermal conversion applications.
Graphical Abstract








Similar content being viewed by others
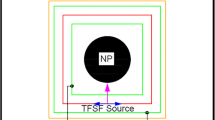
References
Nagar S, Sharma K (2021) Modern solar systems driven by nanoparticles-based fatty acids and paraffin wax phase change materials. J Mater Sci 56:4941–4966. https://doi.org/10.1007/s10853-020-05575-6
Pang YS, Zhang JJ, Ma RM, Qu ZG, Lee E, Luo TF (2020) Solar-thermal water evaporation: a review. ACS Energy Lett 5(2):437–456. https://doi.org/10.1021/acsenergylett.9b02611
Ren HY, Tang M, Guan BL, et al (2017) Hierarchical graphene foam for efficient omnidirectional solar-thermal energy conversion. Adv Mater 29(38): 1702590.1–1702590.7. Doi:https://doi.org/10.1002/adma.201702590.
Mahian O, Kolsi L, Amani M et al (2019) Recent advances in modeling and simulation of nanofluid flows-Part II: applications. Phys Rep 791:1–48. https://doi.org/10.1016/j.physrep.2018.11.004
Baffou G, Quidant R (2012) Thermo-plasmonics: using metallic nanostructures as nano-sources of heat. Laser Photon Rev 7(2):171–187. https://doi.org/10.1002/lpor.201200003
Maier SA (2007) Plasmonics: fundamentals and applications. Springer, New York
Wang F, Shen YR (2006) General properties of local plasmons in metal nanostructures. Phys Rev Lett 97(20):206806.1–206806.4. Doi:https://doi.org/10.1103/PhysRevLett.97.206806.
Chen MJ, He YR, Huang J, Zhu JQ (2016) Synthesis and solar photo-thermal conversion of Au, Ag, and Au-Ag blended plasmonic nanoparticles. Energy Conv Manag 127:293–300. https://doi.org/10.1016/j.enconman.2016.09.015
Qi C, Luo T, Liu M, Fan F, Yan YY (2019) Experimental study on the flow and heat transfer characteristics of nanofluids in double-tube heat exchangers based on thermal efficiency assessment. Energy Conv Manag 197:111877.1–111877.27. Doi:https://doi.org/10.1016/j.enconman.2019.111877.
Wang CY, Zhao JL, Du XH et al (2021) Hydrogen production from ammonia borane hydrolysis catalyzed by non-noble metal-based materials: a review. J Mater Sci 56:2856–2878. https://doi.org/10.1007/s10853-020-05493-7
Qi C, Li KA, Li CY, Shang BC, Yan YY (2020) Experimental study on thermal efficiency improvement using nanofluids in heat sink with heated circular cylinder. Int Commun Heat Mass Transf 114:104589.1–104589.21. Doi:https://doi.org/10.1016/j.icheatmasstransfer.2020.104589.
Chen MJ, He YR, Wang XZ, Hu YW (2018) Numerically investigating the optical properties of plasmonic metallic nanoparticles for effective solar absorption and heating. Sol Energy 161:17–24. https://doi.org/10.1016/j.solener.2017.12.032
Ishii S, Sugavaneshwar RP, Nagao T (2016) Titanium nitride nanoparticles as plasmonic solar heat transducers. J Phys Chem C 120(4):2343–2348. https://doi.org/10.1021/acs.jpcc.5b09604
Qin CY, Kim JB, Gonome H, Lee BJ (2020) Absorption characteristics of nanoparticles with sharp edges for a direct-absorption solar collector. Renew Energ 145:21–28. https://doi.org/10.1016/j.renene.2019.05.133
Wang ZL, Quan XJ, Zhang ZM, Cheng P (2018) Optical absorption of carbon-gold core-shell nanoparticles. J Quant Spectrosc Radiat Transf 205:291–298. https://doi.org/10.1016/j.jqsrt.2017.08.001
Chen XY, Zhou P, Yan HJ, Chen MJ (2021) Systematically investigating solar absorption performance of plasmonic nanoparticles. Energy 216: 119254.1–119254.9. Doi:https://doi.org/10.1016/j.energy.2020.119254.
Chen MJ, He YR, Huang J, Zhu JQ (2016) Synthesis and solar photo-thermal conversion of Au, Ag, and Au–Ag blended plasmonic nanoparticles. Energy Conv Manag 127:293–300. https://doi.org/10.1016/j.enconman.2016.09.015
Du M, Tang GH. Plasmonic nanofluids based on gold nanorods/nanoellipsoids/nanosheets for solar energy harvesting. Sol Energy 137(1):393–400. Doi:https://doi.org/10.1016/j.solener.2016.08.029.
Chen MJ, Wang XZ, Hu YW, He YR (2020) Coupled plasmon resonances of Au thorn nanoparticles to enhance solar absorption performance. J Quant Spectrosc and Radiat Transf 250:107029.1–107029.9. Doi:https://doi.org/10.1016/j.jqsrt.2020.107029.
Chen Z, Chen MJ, Yan HJ, Zhou P, Chen XY (2020) Enhanced solar thermal conversion performance of plasmonic gold dimer nanofluids. Appl Therm Eng 178:115561.1–115561.9. Doi:https://doi.org/10.1016/j.applthermaleng.2020.115561.
Magdassi S, Grouchko M, Kamyshny A (2010) Copper nanoparticles for printed electronics: routes towards achieving oxidation stability. Materials 3(9):4626–4638. https://doi.org/10.3390/ma3094626
Nielsen ND, Smitshuysen TEL, Damsgaard CD, Jensen AD, Christensen JM (2021) Characterization of oxide-supported Cu by infrared measurements on adsorbed CO. Surf Sci 703:121725.1–121725.14. Doi:https://doi.org/10.1016/j.susc.2020.121725.
Jain PK, Lee KS, EI-Sayed IH, EI-Sayed MA (2006) Calculated absorption and scattering properties of gold nanoparticles of different size, shape, and composition: applications in biological imaging and biomedicine. J Phys Chem B 110(14):7238–7248. https://doi.org/10.1021/jp057170o
Liu XL, Xuan YM (2017) Defects-assisted solar absorption of plasmonic nanoshell-based nanofluids. Sol Energy 146:503–510. https://doi.org/10.1016/j.solener.2017.03.024
Wang XJ, Flicker JD, Lee BJ, Ready WJ, Zhang ZM (2009) Visible and near-infrared radiative properties of vertically aligned multi-walled carbon nanotubes. Nanotechnology 20:215704.1–215704.9. Doi:https://doi.org/10.1088/0957-4484/20/21/215704.
Lim DK, Barhoumi A, Wylie RG, Reznor G, Langer RS, Kohane DS (2013) Enhanced photothermal effect of plasmonic nanoparticles coated with reduced graphene oxide. Nano Lett 13(9):4075–4079. https://doi.org/10.1021/nl4014315
Vakili M, Hosseinalipour SM, Delfani S, Khosrojerdi S (2016) Photothermal properties of graphene nanoplatelets nanofluid for low-temperature direct absorption solar collectors. Sol Energy Mater Sol Cells 152:187–191. https://doi.org/10.1016/j.solmat.2016.01.038
Li HB, Kang WJ, ** BJ, Yan Y, Bi HY, Zhu YC, Qian YT (2009) Thermal synthesis of Cu@carbon spherical core–shell structures from carbonaceous matrices containing embedded copper particles. Carbon 48(2):464–469. https://doi.org/10.1016/j.carbon.2009.09.063
Xu YC, Deng LW, Kuang DT, **e HP, Shan DY, Wang X, Sheng XH, Luo H (2020) Cu@C core-shell nanoparticles with efficient optical absorption: DDA-based simulation and experimental validation. Results Phys 16:102885.1–102885.7. Doi:https://doi.org/10.1016/j.rinp.2019.102885.
Chen Z, Chen MJ, Yan HJ, Zhou P, Chen XY (2020) Enhanced solar thermal conversion performance of plasmonic gold dimer nanofluids. Appl Therm Eng 178:115561.1−115561.9. Doi:https://doi.org/10.1016/j.applthermaleng.2020.115561.
Qin CY, Kang K, Lee I, Lee BJ (2017) Optimization of a direct absorption solar collector with blended plasmonic nanofluids. Sol Energy 150:512–520. https://doi.org/10.1016/j.solener.2017.05.007
Zhao J, Pinchuk AO, Mcmahon JM, Li SZ, Ausman LK, Atkinson AL, Schatz GC (2016) Methods for describing the electromagnetic properties of silver and gold nanoparticles. Acc Che Res 41(12):1710–1720. https://doi.org/10.1021/ar800028j
Chen MJ, He YR, Wang XZ, Hu YW (2018) Complementary enhanced solar thermal conversion performance of core-shell nanoparticles. Appl Energy 211:735–742. https://doi.org/10.1016/j.apenergy.2017.11.087
Johnson PB, Christy RW (1974) Optical constants of transition metals: Ti, V, Cr, Mn, Fe Co, Ni, and Pd. Phys Rev B 9(12):5056–5070. https://doi.org/10.1021/ar800028j
Querry MR (1985) Optical constants. Contractor report CRDC-CR-85034, Kansas City.
** JM (1998) Electromagnetic finite element method. ** Dian University Press, **’an.
Tien CL (1988) Thermal radiation in packed and fluidized beds. J Heat Transfer 110(4b):1230–1242. https://doi.org/10.1115/1.3250623
Modest MF (2013) Radiative heat transfer. Academic Press, San Diego
Howell JR, Siegel R, Menguc MP (2010) Thermal radiation heat transfer. CRC Press, New York
Bohren CF, Huffman DR (1998) Absorption and scattering of light by small particles. Wiley, New York
Melnikova IN, Dlugach ZM, Nakajima T, Kawamoto K (2000) Calculation of the reflection function of an optically thick scattering layer for a Henyey-Greenstein phase function. Appl Opt 39(24):4195–4204. https://doi.org/10.1364/AO.39.004195
Ma L, Yu BW, Wang SL, Su G, Huang H, Chen H, He YH, Zou J (2014) Controlled synthesis and optical properties of Cu/C core/shell nanoparticles. J Nanopart Res 16:2545.1–2545.9. Doi:https://doi.org/10.1007/s11051-014-2545-5.
Palik ED (1998) Handbook of optical constants of solids. Academic Press, Maryland
Acknowledgements
This work was financially supported by the Central South University and the National Natural Science Foundation of China (Grant No. 52006246).
Author information
Authors and Affiliations
Corresponding author
Additional information
Handling Editor: Pedro Camargo.
Publisher's Note
Springer Nature remains neutral with regard to jurisdictional claims in published maps and institutional affiliations.
Rights and permissions
About this article
Cite this article
Chen, X., Wu, D., Zhou, P. et al. Modeling the solar absorption performance of Copper@Carbon core–shell nanoparticles. J Mater Sci 56, 13659–13672 (2021). https://doi.org/10.1007/s10853-021-06114-7
Received:
Accepted:
Published:
Issue Date:
DOI: https://doi.org/10.1007/s10853-021-06114-7