Abstract
A search for pair production of scalar leptoquarks, each decaying into either an electron or a muon and a top quark, is presented. This is the first leptoquark search using ATLAS data to investigate top-philic cross-generational couplings that could provide explanations for recently observed anomalies in B meson decays. This analysis targets high leptoquark masses which cause the decay products of each resultant top quark to be contained within a single high-\(p_{\mathrm {T}}\) large-radius jet. The full Run 2 dataset is exploited, consisting of \(139~\hbox {fb}^{-1}\) of data collected from proton–proton collisions at \(\sqrt{s}=13~\mathrm {TeV}\) from 2015 to 2018 with the ATLAS detector at the CERN Large Hadron Collider. In the absence of any significant deviation from the background expectation, lower limits on the leptoquark masses are set at \(1480~\mathrm {GeV}\) and \(1470~\mathrm {GeV}\) for the electron and muon channel, respectively.
Similar content being viewed by others
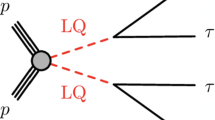
Search for pair production of third-generation leptoquarks decaying into a bottom quark and a $$\tau $$ -lepton with the ATLAS detector
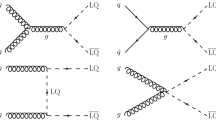
Search for third-generation scalar leptoquarks decaying to a top quark and a $$\tau $$ lepton at $$\sqrt{s}=13\,\text {Te}\text {V} $$
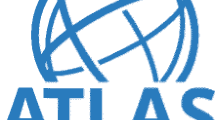
Searches for third-generation scalar leptoquarks in $$ \sqrt{s} $$ = 13 TeV pp collisions with the ATLAS detector
Avoid common mistakes on your manuscript.
1 Introduction
The quark and lepton sectors of the standard model (SM) are interestingly similar, motivating one to hypothesize a fundamental symmetry between the two sectors. Such a symmetry can be found in many grand unified theories, such as grand unified SU(5) [1], the Pati–Salam model based on SU(4) [2], or R-parity-violating (RPV) supersymmetry (SUSY) models [3]. These models predict a new class of bosons carrying both lepton and baryon number, called leptoquarks (LQs). LQs are hypothetical colour-triplet bosons which couple directly to quarks and leptons. They can be of either scalar or vector nature, and carry fractional electric charge. The production cross section of vector LQs could be enhanced relative to that of scalar LQs due to the existence of a massive gluon partner in the minimal set of vector companions [\(\Delta R=0.2\) of a reconstructed electron are removed. If the nearest surviving small-R jet is within \(\Delta R=0.4\) of the electron, then the electron is discarded. To reject hadronic jet candidates produced by bremsstrahlung from very energetic muons, the jet is required to have at least three associated tracks if it lies within a cone of \(\Delta R=0.2\) around a muon candidate. However, if a surviving jet is separated from the nearest muon with transverse momentum \(p^{\mu }_{\mathrm {T}}\) by \(\Delta R < 0.04+10\,\text {Ge}\text {V}/p^{\mu }_{\mathrm {T}}\) up to a maximum of 0.4, the small-R jet is kept and the muon is removed instead; this reduces the background contributions due to muons from hadron decays. No dedicated overlap-removal procedure between large-R and small-R jets is performed. As high-\(p_{\text {T}}\) electrons could deposit significant amounts of energy in the calorimeter to form large-R jets, the electron energy is removed from any overlap** large-R jets before the jet momentum requirements are applied to avoid double counting the electrons as large-R jets. This approach has a 20% better signal efficiency compared to rejecting large-R jets that overlap with a reconstructed electron.
5 Analysis strategy
5.1 Event selection
In the signal region (SR), events were recorded using either a set of single-electron triggers or a set of single-muon triggers. The single-electron triggers imposed a \(p_{\text {T}}\) threshold of 26 \(\text {Ge}\text {V}\) (24 \(\text {Ge}\text {V}\) in 2015) and isolation requirements, or a \(p_{\text {T}}\) threshold of 60 \(\text {Ge}\text {V}\) and no isolation requirements [100] was performed to obtain an unbiased evaluation of the classifier performance. The classifier produces an output score referring to the predicted probability that the event contains LQs, which is then used as the final discriminant to separate LQ signal events from the SM backgrounds.
A natural basis of kinematic observables can be created, utilizing Lorentz symmetry to reduce unnecessary duplication of observables, in the rest frames of intermediate particle states, conditioned on the hypotheses of LQ pair, dileptonic \(t\bar{t}\) or \(Z+\mathrm {jets}\) decay processes. A suite of such discriminating variables is constructed using the recursive jigsaw reconstruction technique [\(p_{\text {T}}\) sum of the two leptons, the two large-R jet masses, and the reconstructed LQ mass. Figure 2 shows the distributions of the dilepton invariant mass in the \(Z+\mathrm {jets}\) CR of the muon channel, and the reconstructed W mass based on a dileptonic \(t\bar{t}\) hypothesis in the \(t\bar{t}\) CR of the electron channel. In general, the kinematic variables show good agreement between data and the background expectation in the CRs. A complete list of the input variables is provided in Table 3.
Distributions of the reconstructed W mass associated with the leading lepton assuming a dileptonic hypothesis in the \(t\bar{t}\) CR after the simultaneous background-only fit of the electron channel CRs (left), and the dilepton invariant mass \(m_{\ell \ell }\) in the \(Z+\mathrm {jets}\) CR after the simultaneous background-only fit of the muon channel CRs (right). The bottom panels show the ratio of data to expected background. The hatched band represents the total uncertainty. The blue triangles indicate points that are outside the vertical range
In order to maximize the sensitivity of the BDT over a wide mass range, and ensure a smooth interpolation of the signal efficiency between the mass points where it was trained, a parameterized machine-learning approach [\(<\,3\%\) on the signal yield.
Other detector-related uncertainties come from uncertainties in the large-R jet mass scales and resolutions; lepton isolation and reconstruction; lepton trigger efficiencies, energy scales, and resolutions; the \(E_{\text {T}}^{\text {miss}}\) reconstruction; pile-up modelling; and the jet-vertex-tagger requirement. Uncertainties in the object momenta are propagated to the \(E_{\text {T}}^{\text {miss}}\) measurement, and additional uncertainties in \(E_{\text {T}}^{\text {miss}}\) arising from the ‘soft’ energy are also considered. These all have negligible impact on the fitted signal yield (<3% each).
6.3 Generator modelling uncertainties
Modelling uncertainties are estimated for the signal as well as Z+jets, \(t\bar{t}\) and single-top-quark backgrounds. The modelling uncertainties are estimated by comparing simulated samples generated with different configurations, described in Sect. 3.
For the LQ signal, in addition to the cross-section uncertainties, the impact on the acceptance due to variations of the QCD scales, PDF and shower parameters was studied. These uncertainties were estimated from the envelope of independent pairs of renormalization and factorization scale variations by a factor of 0.5 and 2, by propagating the PDF and \(\alpha _{\text {S}}\) uncertainties following the PDF4LHC15 prescription, and by considering two alternative samples generated with settings that increase or decrease the amount of QCD radiation. Both the PDF and scale variations have an impact below 15% for all bins considered, while variations of the underlying-event modelling have only a 1–2% effect.
For the Z+jets backgrounds, scale, PDF and \(\alpha _{\text {S}}\) variations are considered and their effects are evaluated within the Sherpa event generator. Seven variations are considered for the renormalization and factorization scales, with the maximum shift within the envelope of those variations taken to estimate the effect of the scale uncertainty. The PDF variations include the variation of the nominal NNPDF3.0nnlo PDF as well as the central values of two other PDF sets, MMHT2014nnlo68cl [Pythia and Herwig, while kee** the same hard-scatter matrix-element calculation. The effects of extra initial- and final-state gluon radiation are estimated by comparing simulated samples generated with enhanced or reduced initial-state radiation, doubling the \(h_\text {damp}\) parameter, and using different values of the radiation parameters [57]. The PDF uncertainty is estimated from the PDF4LHC15 error set. The dominant effect is from the final-state radiation estimation uncertainty and is about 6% of the signal yield.
In this analysis, the single-top-quark background comes mainly from the Wt-channel and is a minor background. Similarly to \(t\bar{t}\) , uncertainties in the hard-scatter generation, the fragmentation and hadronization, the amount of additional radiation, and the PDF are considered. In addition, the uncertainty due to the treatment of the overlap between Wt-channel single top quark production and \(t\bar{t}\) production is considered by comparing samples using the DS and DR methods (see Sect. 3). The dominant effect is from the uncertainty in the fragmentation and hadronization and is about 7% of the signal yield.
7 Statistical interpretation
The binned distributions of the BDT score in the SR and the overall number of events in the \(t\bar{t}\) and \(Z+\mathrm {jets}\) CR are used to test for the presence of a signal. Hypothesis testing is performed using a modified frequentist method as implemented in RooStats [112, 113] and is based on a profile likelihood that takes into account the systematic uncertainties as nuisance parameters that are fitted to the data. A simultaneous fit is performed in the SR and the two CRs, but done separately for the electron and muon channel. As the \(t\bar{t}\) CR is built requiring an electron and muon, the same events are considered in the independent electron and muon channel fits.
The statistical analysis is based on a binned likelihood function \(\mathcal {L}(\mu ,\theta )\) constructed as a product of Poisson probability terms over all bins considered in the search. This function depends on the signal strength parameter \(\mu \), a multiplicative factor applied to the theoretical signal production cross section, and \(\theta \), a set of nuisance parameters that encode the effect of systematic uncertainties in the signal and background expectations and are implemented in the likelihood function as Gaussian and log-normal constraints. Uncertainties in each bin due to the finite size of the simulated samples are also taken into account via dedicated constrained fit parameters. There are enough events in the CRs and the lowest BDT bin in the SR, where the signal contribution is small, to obtain a data-driven estimate of the \(t\bar{t}\) and \(Z+\mathrm {jets}\) normalizations and hence the normalizations of those two backgrounds are included as unconstrained nuisance parameters, \(\mu _{t\bar{t}}\) and \(\mu _{Z}\). Nuisance parameters representing systematic uncertainties are only included in the likelihood if either of the following conditions are met: the overall impact on the normalization in a given region is larger than 3%, or any single bin within the region has at least a 3% uncertainty. This is done separately for each region and for each template (signal or background). When the bin-by-bin statistical variation of a given uncertainty is significant, a smoothing algorithm is applied.
Fit results (background-only) for the binned BDT output score distribution in the signal region of the electron (left) and muon (right) channel, and the overall number of events in the \(t\bar{t}\) and \(Z+\mathrm {jets}\) control regions. The lower panel shows the ratio of data to the fitted background yields. The band represents the systematic uncertainty after the maximum-likelihood fit
The test statistic \(q_{\mu }\) is defined as the profile likelihood ratio, \(q_{\mu }=-2\mathrm {ln}(\mathcal {L}(\mu ,\hat{\hat{\theta }}_{\mu })/ \mathcal {L}(\hat{\mu }, \hat{\theta }))\), where \(\hat{\mu }\) and \(\hat{\theta }\) are the values of the parameters that maximize the likelihood function, and \(\hat{\hat{\theta }}_{\mu }\) are the values of the nuisance parameters that maximize the likelihood function for a given value of \(\mu \). The compatibility of the observed data with the background-only hypothesis is tested by setting \(\mu =0\) in the profile likelihood ratio: \(q_{0}=-2\mathrm {ln}(\mathcal {L}(0,{\hat{\hat{\theta }}}_{0})/\mathcal {L}({\hat{\mu }},{\hat{\theta }}))\). Upper limits on the signal production cross section for each of the signal scenarios considered are derived by using \(q_{\mu }\) in the so-called CL\(_{\mathrm {s}}\) method [114, 115]. For a given signal scenario, values of the production cross section (parameterized by \(\mu \)) yielding CL\(_{\mathrm {s}}<0.05\), where CL\(_{\mathrm {s}}\) is computed using the asymptotic approximation [116], are excluded at \(\ge 95\)% confidence level (CL).
8 Results
8.1 Likelihood fit results
Upper limits at 95% CL on the cross section of LQ pair production as a function of LQ mass, assuming a branching ratio \(\mathcal {B}\) \((\mathrm {LQ} \rightarrow t\ell ^{\pm }) = 1\), for the electron (left) and muon (right) channel. Observed limits are shown as a black solid line and expected limits as a black dashed line. The green and yellow shaded bands correspond to ±1 and ±2 standard deviations, respectively, around the expected limit. The red curve and band show the nominal theoretical prediction and its ±1 standard deviation uncertainty
Lower exclusion limits on the leptoquark mass for scalar leptoquark pair production as a function of the branching ratio into a top quark and an electron (left) or a muon (right) at 95% CL. The observed nominal limits are indicated by a black solid curve, with the surrounding red dotted lines obtained by varying the signal cross section by uncertainties from PDFs, renormalization and factorization scales, and the strong coupling constant \(\alpha _{\text {S}}\). Expected limits are indicated with a black dashed curve, with the yellow and green bands indicating the ±1 standard deviation and ±2 standard deviation excursions due to experimental and modelling uncertainties
The expected and observed event yields in the signal and control regions before and after fitting the background-only hypothesis to data, including all uncertainties, are listed in Table 2. The total uncertainty shown in the table is the uncertainty obtained from the full fit, and is therefore not identical to the sum in quadrature of each component, due to the correlations between the fit parameters. A comparison of the post-fit agreement between data and prediction for the signal and control regions is shown in Fig. 4. In the electron (muon) channel, the ratio of the \(t\bar{t}\) total post-fit yield over the pre-fit yield is \(0.90\pm 0.25\) (\(0.84\pm 0.24\)). The ratio of the \(Z+\mathrm {jets}\) total post-fit yield over the pre-fit yield is \(0.95\pm 0.20\) (\(0.87\pm 0.10\)). None of the individual uncertainties are significantly constrained by data.
The probability that the data is compatible with the background-only hypothesis is estimated by integrating the distribution of the test statistic, approximated using the asymptotic formulae, above the observed value of \(q_{0}\).Footnote 2 This value is computed for each signal scenario considered, defined by the assumed mass of the leptoquark. The lowest local p-value is found to be \(\sim \)11% (10%), for a LQ mass of 1450 (1600) \(\text {Ge}\text {V}\) in the electron (muon) channel. Thus no significant excess above the background expectation is found.
8.2 Limits on LQ pair production
Upper limits at the 95% CL on the LQ pair-production cross section, for an assumed value of \(\beta =1\), are set as a function of the LQ mass \(m_{\mathrm {LQ}}\) and compared with the theoretical prediction (Fig. 5). The resulting lower limit on \(m_{\mathrm {LQ}}\) is determined using the central value of the theoretical NNLO+NNLL cross-section prediction. The observed (expected) lower limits on \(m_{\mathrm {LQ}}\) are found to be 1480 (1560) \(\text {Ge}\text {V}\) and 1470 (1540) \(\text {Ge}\text {V}\) for the electron and muon channel respectively. The sensitivity of the analysis is limited by the statistical uncertainty of the data. Including all systematic uncertainties degrades the expected mass limits by only around 10 \(\text {Ge}\text {V}\), and for a mass of 1.5 \(\text {Te}\text {V}\) the cross-section limits increase by less than 7% in both the electron and muon channel.
Exclusion limits on LQ pair production are also obtained for different values of \(m_{\mathrm {LQ}}\) as a function of the branching ratio (\(\mathcal {B}\)) into a charged lepton and a top quark (Fig. 6). The theoretical cross section was scaled by the branching ratio, and then used to obtain the corresponding limit. The full statistical interpretation is performed for each 0.1 step in \(\mathcal {B}\), covering the full plane.
9 Conclusion
A search for pair production of scalar leptoquarks, each decaying into a top quark and either an electron or a muon has been presented, targeting the high-mass region in which the decay products of each top quark are contained within a single large-radius jet. The analysis is based on tight selection criteria to reduce the SM backgrounds. The normalizations of the dominant \(Z+\mathrm {jets}\) and \(t\bar{t}\) backgrounds were determined simultaneously in a profile likelihood fit to the binned output score of a boosted decision tree in the signal region and two dedicated control regions. The data used in this search correspond to an integrated luminosity of 139 \(\mathrm {fb}^{-1}\) of pp collisions with a centre-of-mass energy \(\sqrt{s}=13~\text {Te}\text {V}\) recorded by the ATLAS experiment in the whole of Run 2 of the LHC. The observed data distributions are compatible with the expected Standard Model background and no significant excess is observed. Lower limits on the leptoquark masses are set at 1480 \(\text {Ge}\text {V}\) and 1470 \(\text {Ge}\text {V}\) for the electron and muon channel, respectively.
Data Availability Statement
This manuscript has no associated data or the data will not be deposited. [Authors’ comment: “All ATLAS scientific output is published in journals, and preliminary results are made available in Conference Notes. All are openly available, without restriction on use by external parties beyond copyright law and the standard conditions agreed by CERN. Data associated with journal publications are also made available: tables and data from plots (e.g. cross section values, likelihood profiles, selection efficiencies, cross section limits, ...) are stored in appropriate repositories such as HEPDATA (http://hepdata.cedar.ac.uk/). ATLAS also strives to make additional material related to the paper available that allows a reinterpretation of the data in the context of new theoretical models. For example, an extended encapsulation of the analysis is often provided for measurements in the framework of RIVET (http://rivet.hepforge.org/).” This information is taken from the ATLAS Data Access Policy, which is a public document that can be downloaded from http://opendata.cern.ch/record/413 [opendata.cern.ch]
Notes
The ATLAS Collaboration uses a right-handed coordinate system with its origin at the nominal interaction point (IP) in the centre of the detector and the z-axis along the beam pipe. The x-axis points from the IP to the centre of the LHC ring, and the y-axis points upwards. Cylindrical coordinates \((r,\phi )\) are used in the transverse plane, \(\phi \) being the azimuthal angle around the beam pipe. The pseudorapidity is defined in terms of the polar angle \(\theta \) as \(\eta =-\ln \tan (\theta /2)\). Angular distance is measured in units of \(\Delta R \equiv \sqrt{(\Delta \eta )^2 + (\Delta \phi )^2} \).
Cross-checks with sampling distributions generated using pseudo-experiments were performed to test the accuracy of the asymptotic approximation for the whole probed leptoquark mass spectrum. The approximation is found to lead to limits that are slightly stronger than those obtained with pseudo-experiments, up to 10% in general for both channels. The impact of this approximation on the mass limits is below 5 \(\text {Ge}\text {V}\).
References
W. Buchmuller, D. Wyler, Constraints on SU(5)-type leptoquarks. Phys. Lett. B 177, 377 (1986). https://doi.org/10.1016/0370-2693(86)90771-9, ISSN: 0370-2693
J.C. Pati, A. Salam, Lepton number as the fourth “color”, Phys. Rev. D 10, 275, (1974) https://doi.org/10.1103/PhysRevD.10.275. Erratum: Phys. Rev. D 11, 703-703 (1975). https://doi.org/10.1103/PhysRevD.11.703.2
R. Barbier et al., R-Parity-violating supersymmetry. Physics Reports 420, 1 (2005). https://doi.org/10.1016/j.physrep.2005.08.006. ISSN: 0370-1573
M.J. Baker, J. Fuentes-Martfn, G. Isidori, M. König, \(p_T\) signatures in vector-leptoquark models. Eur. Phys. J. C 79, 334 (2019). https://doi.org/10.1140/epjc/s10052-019-6853-x. ar**v:1901.10480
BaBar Collaboration, Measurement of an excess of \(\bar{B} \rightarrow D^{(*)}\tau ^{-} \bar{\nu }_\tau \) decays and implications for charged Higgs bosons. Phys. Rev. D 88, 072012 (2013). https://doi.org/10.1103/PhysRevD.88.072012. ar**v:1303.0571
Belle Collaboration, Measurement of the branching ratio of \(\bar{B} \rightarrow D^{(\ast )} \tau ^{-} \bar{\nu }_\tau \) relative to \(\bar{B} \rightarrow D^{(\ast )} \ell ^{-} \bar{\nu }_\ell \) decays with hadronic tagging at Belle. Phys. Rev. D 92, 072014 (2015). https://doi.org/10.1103/PhysRevD.92.072014. ar**v:1507.03233
LHCb Collaboration, Measurement of the Ratio of Branching Fractions \(\cal B\it (\bar{B}^0 \rightarrow D^{*+}\tau ^{-}\bar{\nu }_{\tau })/\cal B\it (\bar{B}^0 \rightarrow D^{*+}\mu ^{-}\bar{\nu }_{\mu })\), Phys. Rev. Lett. 115, 111803, (2015). https://doi.org/10.1103/PhysRevLett.115.111803, ar**v:1506.08614, Erratum: Phys. Rev. Lett. 115, 159901, (2015). https://doi.org/10.1103/PhysRevLett.115.159901
LHCb Collaboration, Test of lepton universality with \(B^{0} \rightarrow K^{*0}\ell ^{+}\ell ^{-}\) decays, JHEP 08, 055, (2017). https://doi.org/10.1007/JHEP08(2017)055, ar**v:1705.05802
LHCb Collaboration, Search for Lepton-Universality Violation in \(B^+\rightarrow K^+\ell ^+\ell ^-\) Decays, Phys. Rev. Lett. 122, 191801, (2019). https://doi.org/10.1103/PhysRevLett.122.191801, ar**v:1903.09252
G. Hiller, D. Loose, I. Nišandžić, Flavorful leptoquarks at hadron colliders. Phys. Rev. D 97, 075004 (2018). https://doi.org/10.1103/PhysRevD.97.075004. ar**v:1801.09399
A.C.J. Camargo-Molina, D. Faroughy, Anomalies in bottom from new physics in top. Phys. Lett. B 784, 284 (2018). https://doi.org/10.1016/j.physletb.2018.07.051. ar**v:1805.04917
C.-H. Chen, T. Nomura, H. Okada, Excesses of muon \(g-2\), \(R_{D^{(\ast )}}\), and \(R_{K^{(\ast )}}\) in a leptoquark model. Phys. Lett. B 774, 456 (2017). https://doi.org/10.1016/j.physletb.2017.10.005. ar**v:1703.03251
Muon g-2 Collaboration, Final report of the E821 muon anomalous magnetic moment measurement at BNL, Phys. Rev. D 73, 072003, (2006). https://doi.org/10.1103/PhysRevD.73.072003, ar**v:hep-ex/0602035
L. Evans, P. Bryant, LHC Machine. JINST 3, S08001 (2008). https://doi.org/10.1088/1748-0221/3/08/S08001
C. Borschensky, B. Fuks, A. Kulesza, D. Schwartländer, Scalar leptoquark pair production at hadron colliders. Phys. Rev. D 101, 115017 (2020). https://doi.org/10.1103/PhysRevD.101.115017. ar**v:2002.08971 [hep-ph]
T. Mandal, S. Mitra, S. Seth, Pair production of scalar leptoquarks at the LHC to NLO parton shower accuracy. Phys. Rev. D 93, 035018 (2016). https://doi.org/10.1103/PhysRevD.93.035018. ar**v:1506.07369 [hep-ph]
M. Kramer, T. Plehn, M. Spira, P. Zerwas, Pair production of scalar leptoquarks at the CERN LHC. Phys. Rev. D 71, 057503 (2005). https://doi.org/10.1103/PhysRevD.71.057503. ar**v:hep-ph/0411038
M. Kramer, T. Plehn, M. Spira, P. Zerwas, Pair Production of Scalar Leptoquarks at the Fermilab Tevatron. Phys. Rev. Lett. 79, 341 (1997). https://doi.org/10.1103/PhysRevLett.79.341. ar**v:hep-ph/9704322
ATLAS Collaboration, Search for pairs of scalar leptoquarks decaying into quarks and electrons or muons in \(\sqrt{s}=13\) TeV pp collisions with the ATLAS detector, (2020). https://doi.org/10.1007/JHEP10(2020)112, ar**v:2006.05872
ATLAS Collaboration, Search for pair production of third-generation scalar leptoquarks decaying into a top quark and a \(\tau \)-lepton in \(pp\) collisions at \(\sqrt{s} = 13\,\text{TeV}\) with the ATLAS detector, (2021). ar**v:2006.05872
R. Mandal, A. Pich, Constraints on scalar leptoquarks from lepton and kaon physics. JHEP 12, 089 (2019). https://doi.org/10.1007/JHEP12(2019)089. ar**v:1908.11155 [hep-ph]
CMS Collaboration, Search for Leptoquarks Coupled to Third-Generation Quarks in Proton-Proton Collisions at \(\sqrt{s} = 13\) TeV, Phys. Rev. Lett. 121, 241802, (2018), https://doi.org/10.1103/PhysRevLett.121.241802, ar**v:1809.05558
ATLAS Collaboration, The ATLAS Experiment at the CERN Large Hadron Collider, JINST 3, S08003, (2008) https://doi.org/10.1088/1748-0221/3/08/S08003
ATLAS Collaboration, ATLAS Insertable B-Layer Technical Design Report, ATLAS-TDR-19; CERN-LHCC-2010-013, 2010, URL: https://cds.cern.ch/record/1291633
B. Abbott et al., Production and integration of the ATLAS Insertable B-Layer. JINST 13, T05008 (2018). https://doi.org/10.1088/1748-0221/13/05/T05008. ar**v:1803.00844 [physics.ins-det]
ATLAS Collaboration, Performance of the ATLAS trigger system in 2015, Eur. Phys. J. C 77, 317, (2017), https://doi.org/10.1140/epjc/s10052-017-4852-3, ar**v:1611.09661 [hep-ex]
J. Alwall et al., The automated computation of tree-level and next-to-leading order differential cross sections, and their matching to parton shower simulations. JHEP 07, 079 (2014). https://doi.org/10.1007/JHEP07(2014)079. ar**v:1405.0301
R.D. Ball et al., Parton distributions for the LHC run II. JHEP 04, 040 (2015). https://doi.org/10.1007/JHEP04(2015)040. ar**v:1410.8849
T. Sjöstrand et al., An introduction to PYTHIA 8.2. Comput. Phys. Commun. 191, 159 (2015). https://doi.org/10.1016/j.cpc.2015.01.024. ar**v:1410.3012
ATLAS Collaboration, ATLAS Pythia 8 tunes to 7 TeV data, tech. rep. ATL-PHYS-PUB-2014-021, CERN, 2014, URL: https://cds.cern.ch/record/1966419
R.D. Ball et al., Parton distributions with LHC data. Nucl. Phys. B 867, 244 (2013). https://doi.org/10.1016/j.nuclphysb.2012.10.003. ar**v:1207.1303 [hep-ph]
L. Lönnblad, S. Prestel, Merging multi-leg NLO matrix elements with parton showers. JHEP 03, 166 (2013). https://doi.org/10.1007/JHEP03(2013)166. ar**v:1211.7278 [hep-ph]
W. Beenakker, C. Borschensky, M. Krämer, A. Kulesza, E. Laenen, NNLL-fast: predictions for coloured supersymmetric particle production at the LHC with threshold and Coulomb resummation. JHEP 12, 133 (2016). https://doi.org/10.1007/JHEP12(2016)133. ar**v:1607.07741 [hep-ph]
W. Beenakker, M. Kramer, T. Plehn, M. Spira, P. Zerwas, Stop production at hadron colliders. Nucl. Phys. B 515, 3 (1998). https://doi.org/10.1016/S0550-3213(98)00014-5. ar**v:hep-ph/9710451
W. Beenakker et al., Supersymmetric top and bottom squark production at hadron colliders. JHEP 08, 098 (2010). https://doi.org/10.1007/JHEP08(2010)098. ar**v:1006.4771 [hep-ph]
W. Beenakker et al., NNLL resummation for stop pair-production at the LHC. JHEP 05, 153 (2016). https://doi.org/10.1007/JHEP05(2016)153. ar**v:1601.02954 [hep-ph]
S. Frixione, E. Laenen, P. Motylinski, B.R. Webber, Angular correlations of lepton pairs from vector boson and top quark decays in Monte Carlo simulations. JHEP 04, 081 (2007). https://doi.org/10.1088/1126-6708/2007/04/081. ar**v:hep-ph/0702198
P. Artoisenet, R. Frederix, O. Mattelaer, R. Rietkerk, Automatic spin-entangled decays of heavy resonances in Monte Carlo simulations. JHEP 03, 015 (2013). https://doi.org/10.1007/JHEP03(2013)015. ar**v:1212.3460
E. Bothmann et al., Event generation with Sherpa 2.2. SciPost Phys. 7, 034 (2019). https://doi.org/10.21468/SciPostPhys.7.3.034. ar**v:1905.09127
C. Anastasiou, L.J. Dixon, K. Melnikov, F. Petriello, High-precision QCD at hadron colliders: Electroweak gauge boson rapidity distributions at next-to-next-to leading order. Phys. Rev. D 69, 094008 (2004). https://doi.org/10.1103/PhysRevD.69.094008. ar**v:hep-ph/0312266
T. Gleisberg, S. Höche, Comix, a new matrix element generator. JHEP 12, 039 (2008). https://doi.org/10.1088/1126-6708/2008/12/039. ar**v:0808.3674
F. Buccioni et al., OpenLoops 2. Eur. Phys. J. C 79, 866 (2019). https://doi.org/10.1140/epjc/s10052-019-7306-2. ar**v:1907.13071
F. Cascioli, P. Maierhöfer, S. Pozzorini, Scattering Amplitudes with Open Loops. Phys. Rev. Lett. 108, 111601 (2012). https://doi.org/10.1103/PhysRevLett.108.111601. ar**v:1111.5206
A. Denner, S. Dittmaier, L. Hofer, COLLIER: A fortran-based complex one-loop library in extended regularizations. Comput. Phys. Commun. 212, 220 (2017). https://doi.org/10.1016/j.cpc.2016.10.013. ar**v:1604.06792
S. Schumann, F. Krauss, A parton shower algorithm based on Catani-Seymour dipole factorisation. JHEP 03, 038 (2008). https://doi.org/10.1088/1126-6708/2008/03/038. ar**v:0709.1027
S. Höche, F. Krauss, M. Schönherr, F. Siegert, A critical appraisal ofNLO+PS matching methods. JHEP 09, 049 (2012). https://doi.org/10.1007/JHEP09(2012)049. ar**v:1111.1220
S. Höche, F. Krauss, M. Schönherr, F. Siegert, QCD matrix elements + parton showers. The NLO case, JHEP 04, 027 (2013). https://doi.org/10.1007/JHEP04(2013)027. ar**v:1207.5030
S. Catani, F. Krauss, R. Kuhn, B.R. Webber, QCD Matrix Elements + Parton Showers. JHEP 11, 063 (2001). https://doi.org/10.1088/1126-6708/2001/11/063. ar**v:hep-ph/0109231
S. Höche, F. Krauss, S. Schumann, F. Siegert, QCD matrix elements and truncated showers. JHEP 05, 053 (2009). https://doi.org/10.1088/1126-6708/2009/05/053. ar**v:0903.1219
P. Nason, A new method for combining NLO QCD with shower Monte Carlo algorithms. JHEP 11, 040 (2004). https://doi.org/10.1088/1126-6708/2004/11/040. ar**v:hep-ph/0409146
S. Frixione, P. Nason, C. Oleari, Matching NLO QCD computations with parton shower simulations: the POWHEG method. JHEP 11, 070 (2007). https://doi.org/10.1088/1126-6708/2007/11/070. ar**v:0709.2092
S. Alioli, P. Nason, C. Oleari, E. Re, A general framework for implementing NLO calculations in shower Monte Carlo programs: the POWHEG BOX. JHEP 06, 043 (2010). https://doi.org/10.1007/JHEP06(2010)043. ar**v:1002.2581
S. Frixione, P. Nason, G. Ridolfi, A positive-weight next-to-leading-order Monte Carlo for heavy flavour hadroproduction. JHEP 09, 126 (2007). https://doi.org/10.1088/1126-6708/2007/09/126. ar**v:0707.3088
E. Re, Single-top Wt-channel production matched with parton showers using the POWHEG method. Eur. Phys. J. C 71, 1547 (2011). https://doi.org/10.1140/epjc/s10052-011-1547-z. ar**v:1009.2450
S. Alioli, P. Nason, C. Oleari and E. Re, NLO single-top production matched with shower in POWHEG: s- and t-channel contributions, JHEP 09, 111, (2009). https://doi.org/10.1088/1126-6708/2009/09/111, ar**v:0907.4076, Erratum: JHEP 02, 011, (2010). https://doi.org/10.1007/JHEP02(2010)011
S. Frixione, E. Laenen, P. Motylinski, B.R. Webber, C.D. White, Single-top hadroproduction in association with a W boson. JHEP 07, 029 (2008). https://doi.org/10.1088/1126-6708/2008/07/029. ar**v:0805.3067
ATLAS Collaboration, Studies on top-quark Monte Carlo modelling for Top2016, ATL-PHYS-PUB-2016-020, 2016, URL: https://cds.cern.ch/record/2216168
M. Beneke, P. Falgari, S. Klein, C. Schwinn, Hadronic top-quark pair production with NNLL threshold resummation. Nucl. Phys. B 855, 695 (2012). https://doi.org/10.1016/j.nuclphysb.2011.10.021. ar**v:1109.1536
M. Cacciari, M. Czakon, M. Mangano, A. Mitov, P. Nason, Top-pair production at hadron colliders with next-to-next-to-leading logarithmic soft-gluon resummation. Phys. Lett. B 710, 612 (2012). https://doi.org/10.1016/j.physletb.2012.03.013. ar**v:1111.5869
P. Bärnreuther, M. Czakon, A. Mitov, Percent-Level-Precision Physics at the Tevatron: Next-to-Next-to-Leading Order QCD Corrections to \(q \bar{q} \rightarrow t \bar{t} + X\). Phys. Rev. Lett. 109, 132001 (2012). https://doi.org/10.1103/PhysRevLett.109.132001. ar**v:1204.5201
M. Czakon, A. Mitov, NNLO corrections to top-pair production at hadron colliders: the all-fermionic scattering channels. JHEP 12, 054 (2012). https://doi.org/10.1007/JHEP12(2012)054. ar**v:1207.0236
M. Czakon, A. Mitov, NNLO corrections to top pair production at hadron colliders: the quark-gluon reaction. JHEP 01, 080 (2013). https://doi.org/10.1007/JHEP01(2013)080. ar**v:1210.6832
M. Czakon, P. Fiedler, A. Mitov, Total Top-Quark Pair-Production Cross Section at Hadron Colliders Through \(O(\alpha _S^4)\). Phys. Rev. Lett. 110, 252004 (2013). https://doi.org/10.1103/PhysRevLett.110.252004. ar**v:1303.6254
M. Czakon, A. Mitov, Top++: A program for the calculation of the top-pair cross-section at hadron colliders. Comput. Phys. Commun. 185, 2930 (2014). https://doi.org/10.1016/j.cpc.2014.06.021. ar**v:1112.5675
M. Aliev et al., HATHOR - HAdronic Top and Heavy quarks crOss section calculatoR. Comput. Phys. Commun. 182, 1034 (2011). https://doi.org/10.1016/j.cpc.2010.12.040. ar**v:1007.1327
P. Kant et al., HATHOR for single top-quark production: Updated predictions and uncertainty estimates for single top-quark production in hadronic collisions. Comput. Phys. Commun. 191, 74 (2015). https://doi.org/10.1016/j.cpc.2015.02.001. ar**v:1406.4403
N. Kidonakis, Two-loop soft anomalous dimensions for single top quark associated production with a \(W^{-}\) or \(H^{-}\). Phys. Rev. D 82, 054018 (2010). https://doi.org/10.1103/PhysRevD.82.054018. ar**v:1005.4451 [hep-ph]
N. Kidonakis, ’Top Quark Production’, Proceedings, Helmholtz International Summer School on Physics of Heavy Quarks and Hadrons (HQ 2013): JINR, Dubna, Russia, July 15-28, 2013, 2014 139, https://doi.org/10.3204/DESY-PROC-2013-03/Kidonakis, ar**v:1311.0283 [hep-ph]
M. Bahr et al., Herwig++ physics and manual. Eur. Phys. J. C 58, 639 (2008). https://doi.org/10.1140/epjc/s10052-008-0798-9. ar**v:0803.0883
J. Bellm et al., Herwig 7.0/Herwig++ 3.0 release note, Eur. Phys. J. C 76 (2016) 196, 10.1140/epjc/s10052-016-4018-8, ar**v:1512.01178
D. de Florian et al., Handbook of LHC Higgs Cross Sections: 4. Deciphering the Nature of the Higgs Sector, (2016), https://doi.org/10.23731/CYRM-2017-002, ar**v:1610.07922
D.J. Lange, The EvtGen particle decay simulation package. Nucl. Instrum. Meth. A 462, 152 (2001). https://doi.org/10.1016/S0168-9002(01)00089-4
ATLAS Collaboration, The ATLAS Simulation Infrastructure, Eur. Phys. J. C 70, 823, (2010), https://doi.org/10.1140/epjc/s10052-010-1429-9, ar**v:1005.4568 [physics.ins-det]
GEANT4 Collaboration, S. Agostinelli et al., GEANT4 - a simulation toolkit, Nucl. Instrum. Meth. A 506, 250, (2003), https://doi.org/10.1016/S0168-9002(03)01368-8
ATLAS Collaboration, The simulation principle and performance of the ATLAS fast calorimeter simulation FastCaloSim, ATL-PHYS-PUB-2010-013 (2010), URL: https://cds.cern.ch/record/1300517
T. Sjöstrand, S. Mrenna and P. Skands, A brief introduction to PYTHIA 8.1, Comput. Phys. Commun. 178 (2008) 852. https://doi.org/10.1016/j.cpc.2008.01.036. ar**v:0710.3820
ATLAS Collaboration, The Pythia 8 A3 tune description of ATLAS minimum bias and inelastic measurements incorporating the Donnachie-Landshoff diffractive model, ATL-PHYS-PUB-2016-017, 2016, URL: https://cds.cern.ch/record/2206965
ATLAS Collaboration, Electron and photon performance measurements with the ATLAS detector using the 2015-2017 LHC proton-proton collision data, JINST 14 (2019) P12006. https://doi.org/10.1088/1748-0221/14/12/P12006, ar**v:1908.00005
ATLAS Collaboration, Muon reconstruction performance of the ATLAS detector in proton-proton collision data at \(\sqrt{s}\) =13 TeV, Eur. Phys. J. C 76, 292, (2016). https://doi.org/10.1140/epjc/s10052-016-4120-y, ar**v:1603.05598
M. Cacciari, G.P. Salam, G. Soyez, The anti-\(k_{t}\) jet clustering algorithm. JHEP 04, 063 (2008). https://doi.org/10.1088/1126-6708/2008/04/063. ar**v:0802.1189
M. Cacciari, G.P. Salam, G. Soyez, FastJet user manual. Eur. Phys. J. C 72, 1896 (2012). https://doi.org/10.1140/epjc/s10052-012-1896-2. ar**v:1111.6097 [hep-ph]
ATLAS Collaboration, Jet reconstruction and performance using particle flow with the ATLAS Detector, Eur. Phys. J. C 77, 466, (2017), https://doi.org/10.1140/epjc/s10052-017-5031-2, ar**v:1703.10485
ATLAS Collaboration, Jet energy scale and resolution measured in proton-proton collisions at \(\sqrt{s}=13~\text{ TeV }\) with the ATLAS detector, (2020), ar**v:2007.02645
ATLAS Collaboration, Topological cell clustering in the ATLAS calorimeters and its performance in LHC Run 1, Eur. Phys. J. C 77, 490, (2017), https://doi.org/10.1140/epjc/s10052-017-5004-5, ar**v:1603.02934 [hep-ex]
ATLAS Collaboration, Performance of pile-up mitigation techniques for jets in \(pp\) collisions at \(\sqrt{s}=8\) TeV using the ATLAS detector, Eur. Phys. J. C 76 (2016) 581, https://doi.org/10.1140/epjc/s10052-016-4395-z, ar**v:1510.03823
D. Krohn, J. Thaler, L.-T. Wang, Jet trimming. JHEP 02, 084 (2010). https://doi.org/10.1007/JHEP02(2010)084. ar**v:0912.1342
S. Catani, Y.L. Dokshitzer, M. Olsson, G. Turnock, B. Webber, New clustering algorithm for multijet cross-sections in e+ e- annihilation. Phys. Lett. B 269, 432 (1991). https://doi.org/10.1016/0370-2693(91)90196-W
S.D. Ellis, D.E. Soper, Successive combination jet algorithm for hadron collisions. Phys. Rev. D 48, 3160 (1993). https://doi.org/10.1103/PhysRevD.48.3160. ar**v:hep-ph/9305266
S. Catani, Y.L. Dokshitzer, M. Seymour, B. Webber, Longitudinally-invariant \(k_{\perp }\)-clustering algorithms for hadron-hadron collisions. Nucl. Phys. B 406, 187 (1993). https://doi.org/10.1016/0550-3213(93)90166-M
ATLAS Collaboration, Performance of jet substructure techniques for large-\(R\) jets in proton-proton collisions at \(\sqrt{s} = 7\,\text{ TeV }\) using the ATLAS detector, JHEP 09, 076, (2013), https://doi.org/10.1007/JHEP09(2013)076, ar**v:1306.4945 [hep-ex]
J. Thaler, K.V. Tilburg, Identifying boosted objects with N-subjettiness. JHEP 03, 015 (2011). https://doi.org/10.1007/JHEP03(2011)015. ar**v:1011.2268
J. Thaler, K.V. Tilburg, Maximizing boosted top identification by minimizing N-subjettiness. JHEP 02, 093 (2012). https://doi.org/10.1007/JHEP02(2012)093. ar**v:1108.2701
ATLAS Collaboration, Measurement of \(k_T\) splitting scales in \(W \rightarrow l\nu \) events at \(\sqrt{s}\)=7 TeV with the ATLAS detector, Eur. Phys. J. C 73, 2432, (2013). https://doi.org/10.1140/epjc/s10052-013-2432-8, ar**v:1302.1415
J. Thaler, L.-T. Wang, Strategies to identify boosted tops. JHEP 07, 092 (2008). https://doi.org/10.1088/1126-6708/2008/07/092. ar**v:0806.0023
ATLAS Collaboration, Performance of missing transverse momentum reconstruction with the ATLAS detector using proton–proton collisions at \(\sqrt{s} = 13\,\text{ TeV }\), Eur. Phys. J. C 78, 903, (2018), https://doi.org/10.1140/epjc/s10052-018-6288-9, ar**v:1802.08168 [hep-ex]
ATLAS Collaboration, \(E_{\text{ T }}^{\text{ miss }}\) performance in the ATLAS detector using 2015-2016 LHC pp collisions, ATLAS-CONF-2018-023, 2018, URL: https://cds.cern.ch/record/2625233
ATLAS Collaboration, Performance of electron and photon triggers in ATLAS during LHC Run 2, Eur. Phys. J. C 80, 47, (2020). https://doi.org/10.1140/epjc/s10052-019-7500-2, ar**v:1909.00761 [hep-ex]
ATLAS Collaboration, Performance of the ATLAS muon triggers in Run 2, (2020). https://doi.org/10.1088/1748-0221/15/09/p09015, ar**v:2004.13447 [hep-ex]
T. Chen, C. Guestrin, XGBoost: A Scalable Tree Boosting System, (2016), https://doi.org/10.1145/2939672.2939785, ar**v:1603.02754
M. Stone, Cross-Validatory Choice and Assessment of Statistical Predictions. J. Roy. Stat. Soc. B 36, 111 (1974). https://doi.org/10.1111/j.2517-6161.1974.tb00994.x
P. Jackson, C. Rogan, Recursive jigsaw reconstruction: HEP event analysis in the presence of kinematic and combinatoric ambiguities. Phys. Rev. D 96, 112007 (2017). https://doi.org/10.1103/PhysRevD.96.112007. ar**v:1705.10733
P. Baldi, K. Cranmer, T. Faucett, P. Sadowski, D. Whiteson, Parameterized neural networks for high-energy physics. Eur. Phys. J. C 76, 235 (2016). https://doi.org/10.1140/epjc/s10052-016-4099-4. ar**v:1601.07913 [hep-ex]
ATLAS Collaboration, Luminosity determination in \(pp\) collisions at \(\sqrt{s} = 13\,\text{ TeV }\) using the ATLAS detector at the LHC, ATLAS-CONF-2019-021, 2019, URL: https://cds.cern.ch/record/2677054
G. Avoni et al., The new LUCID-2 detector for luminosity measurement and monitoring in ATLAS, JINST 13, P07017, (2018). https://doi.org/10.1088/1748-0221/13/07/P07017
J. Butterworth et al., PDF4LHC recommendations for LHC Run II. J. Phys. G 43, 023001 (2016). https://doi.org/10.1088/0954-3899/43/2/023001. ar**v:1510.03865
K. Melnikov, F. Petriello, Electroweak gauge boson production at hadron colliders through \(O(\alpha _s^2)\). Phys. Rev. D 74, 114017 (2006). https://doi.org/10.1103/PhysRevD.74.114017. ar**v:hep-ph/0609070
ATLAS Collaboration, Multi-boson simulation for 13 TeV ATLAS analyses, ATL-PHYS-PUB-2016-002, 2016, URL: https://cds.cern.ch/record/2119986
ATLAS Collaboration, Modelling of the \(t\bar{t} H\) and \(t\bar{t}V (V = W, Z)\) processes for \(\sqrt{s} = 13~\text{ TeV }\) ATLAS analyses, ATL-PHYS-PUB-2016-005, 2016, URL: https://cds.cern.ch/record/2120826
ATLAS Collaboration, Jet Calibration and Systematic Uncertainties for Jets Reconstructed in the ATLAS Detector at \(\sqrt{s} = 13\) TeV, ATL-PHYS-PUB-2015-015 (2015), URL: https://cds.cern.ch/record/2037613
L. Harland-Lang, A. Martin, P. Motylinski, R. Thorne, Parton distributions in the LHC era: MMHT 2014 PDFs. Eur. Phys. J. C 75, 204 (2015). https://doi.org/10.1140/epjc/s10052-015-3397-6. ar**v:1412.3989
S. Dulat et al., New parton distribution functions from a global analysis ofquantum chromodynamics. Phys. Rev. D 93, 033006 (2016). https://doi.org/10.1103/PhysRevD.93.033006. ar**v:1506.07443
W. Verkerke, D. Kirkby, The RooFit toolkit for data modeling, (2003), ar**v:physics/0306116
W. Verkerke, D. Kirkby, RooFit Users Manual v2.91, (2008), URL: http://roofit.sourceforge.net
A.L. Read, Presentation of search results: the \(CL_s\) technique. J. Phys. G 28, 2693 (2002). https://doi.org/10.1088/0954-3899/28/10/313
T. Junk, Confidence level computation for combining searches with small statistics. Nucl. Instrum. Meth. A 434, 435 (1999). https://doi.org/10.1016/S0168-9002(99)00498-2. ar**v:hep-ex/9902006
G. Cowan, K. Cranmer, E. Gross, O. Vitells, Asymptotic formulae for likelihood-based tests of new physics, Eur. Phys. J. C 71, 1554, (2011), https://doi.org/10.1140/epjc/s10052-011-1554-0, ar**v:1007.1727, Erratum: Eur. Phys. J. C 73, 2501, (2013). https://doi.org/10.1140/epjc/s10052-013-2501-z
A.J. Larkoski, D. Neill, J. Thaler, Jet shapes with the broadening axis. JHEP 04, 017 (2014). https://doi.org/10.1007/JHEP04(2014)017. ar**v:1401.2158 [hep-ph]
ATLAS Collaboration, ATLAS Computing Acknowledgements, ATL-SOFT-PUB-2020-001, URL: https://cds.cern.ch/record/2717821
Acknowledgements
We thank CERN for the very successful operation of the LHC, as well as the support staff from our institutions without whom ATLAS could not be operated efficiently.
We acknowledge the support of ANPCyT, Argentina; YerPhI, Armenia; ARC, Australia; BMWFW and FWF, Austria; ANAS, Azerbaijan; SSTC, Belarus; CNPq and FAPESP, Brazil; NSERC, NRC and CFI, Canada; CERN; ANID, Chile; CAS, MOST and NSFC, China; COLCIENCIAS, Colombia; MSMT CR, MPO CR and VSC CR, Czech Republic; DNRF and DNSRC, Denmark; IN2P3-CNRS and CEA-DRF/IRFU, France; SRNSFG, Georgia; BMBF, HGF and MPG, Germany; GSRT, Greece; RGC and Hong Kong SAR, China; ISF and Benoziyo Center, Israel; INFN, Italy; MEXT and JSPS, Japan; CNRST, Morocco; NWO, Netherlands; RCN, Norway; MNiSW and NCN, Poland; FCT, Portugal; MNE/IFA, Romania; JINR; MES of Russia and NRC KI, Russian Federation; MESTD, Serbia; MSSR, Slovakia; ARRS and MIZŠ, Slovenia; DST/NRF, South Africa; MICINN, Spain; SRC and Wallenberg Foundation, Sweden; SERI, SNSF and Cantons of Bern and Geneva, Switzerland; MOST, Taiwan; TAEK, Turkey; STFC, United Kingdom; DOE and NSF, United States of America. In addition, individual groups and members have received support from BCKDF, CANARIE, Compute Canada, CRC and IVADO, Canada; Bei**g Municipal Science & Technology Commission, China; COST, ERC, ERDF, Horizon 2020 and Marie Skłodowska-Curie Actions, European Union; Investissements d’Avenir Labex, Investissements d’Avenir Idex and ANR, France; DFG and AvH Foundation, Germany; Herakleitos, Thales and Aristeia programmes co-financed by EU-ESF and the Greek NSRF, Greece; BSF-NSF and GIF, Israel; La Caixa Banking Foundation, CERCA Programme Generalitat de Catalunya and PROMETEO and GenT Programmes Generalitat Valenciana, Spain; Göran Gustafssons Stiftelse, Sweden; The Royal Society and Leverhulme Trust, United Kingdom.
The crucial computing support from all WLCG partners is acknowledged gratefully, in particular from CERN, the ATLAS Tier-1 facilities at TRIUMF (Canada), NDGF (Denmark, Norway, Sweden), CC-IN2P3 (France), KIT/GridKA (Germany), INFN-CNAF (Italy), NL-T1 (Netherlands), PIC (Spain), ASGC (Taiwan), RAL (UK) and BNL (USA), the Tier-2 facilities worldwide and large non-WLCG resource providers. Major contributors of computing resources are listed in Ref. [118].
Author information
Authors and Affiliations
Department of Physics, University of Adelaide, Adelaide, Australia
D. Duvnjak, P. Jackson, A. X. Y. Kong, J. L. Oliver, T. A. Ruggeri, A. S. Sharma & M. J. White
Physics Department, SUNY Albany, Albany, NY, USA
V. Jain & S. P. Swift
Department of Physics, University of Alberta, Edmonton, AB, Canada
J. P. Biswal, D. M. Gingrich, J. L. Pinfold & H. Wang
Department of Physics, Ankara University, Ankara, Turkey
O. Cakir & H. Duran Yildiz
Istanbul Aydin University, Application and Research Center for Advanced Studies, Istanbul, Turkey
S. Kuday & I. Turk Cakir
Division of Physics, TOBB University of Economics and Technology, Ankara, Turkey
S. Sultansoy
LAPP, Université Grenoble Alpes, Université Savoie Mont Blanc, CNRS/IN2P3, Annecy, France
C. Adam Bourdarios, M. Belfkir, N. Berger, F. Costanza, A. Cueto, O. Dartsi, M. Delmastro, L. Di Ciaccio, L. Franco, C. Goy, T. Guillemin, T. Hryn’ova, S. Jézéquel, I. Koletsou, R. Lafaye, J. Levêque, N. Lorenzo Martinez, L. Portales, E. Sauvan & I. Wingerter-Seez
High Energy Physics Division, Argonne National Laboratory, Argonne, IL, USA
Y. Abulaiti, D. P. Benjamin, V. S. Bhopatkar, S. Chekanov, W. H. Hopkins, E. Kourlitis, T. LeCompte, J. Love, J. Metcalfe, A. S. Mete, A. Paramonov, J. Proudfoot, P. Van Gemmeren, R. Wang & J. Zhang
Department of Physics, University of Arizona, Tucson, AZ, USA
S. Berlendis, E. Cheu, C. M. Delitzsch, K. A. Johns, W. Lampl, M. LeBlanc, R. E. Lindley, P. Loch, J. P. Rutherfoord, E. W. Varnes, H. Zhou & Y. Zhou
Department of Physics, University of Texas at Arlington, Arlington, TX, USA
D. Bakshi Gupta, B. Burghgrave, K. De, T. Eifert, A. Farbin, H. K. Hadavand, J. D. Little, N. Ozturk, G. Usai & A. White
Physics Department, National and Kapodistrian University of Athens, Athens, Greece
S. Angelidakis, P. Bellos, D. Fassouliotis, I. Gkialas, C. Kourkoumelis & K. Papageorgiou
Physics Department, National Technical University of Athens, Zografou, Greece
T. Alexopoulos, C. Bakalis, N. Benekos, E. N. Gazis, P. Gkountoumis, C. Kitsaki, A. Koulouris, S. Maltezos, C. Paraskevopoulos & G. Zacharis
Department of Physics, University of Texas, Austin, TX, USA
T. Andeen, C. D. Burton, K. Choi, N. Nikiforou, P. U. E. Onyisi, H. Potti, A. Roy, M. Unal & A. F. Webb
Bahcesehir University, Faculty of Engineering and Natural Sciences, Istanbul, Turkey
A. J. Beddall
Istanbul Bilgi University, Faculty of Engineering and Natural Sciences, Istanbul, Turkey
E. Celebi, S. A. Cetin & S. Simsek
Department of Physics, Bogazici University, Istanbul, Turkey
A. Adiguzel, A. Bayirli, S. Gurbuz & V. E. Ozcan
Department of Physics Engineering, Gaziantep University, Gaziantep, Turkey
A. Beddall, A. Bingul & Z. Uysal
Institute of Physics, Azerbaijan Academy of Sciences, Baku, Azerbaijan
F. Khalil-Zada
Institut de Física d’Altes Energies (IFAE), Barcelona Institute of Science and Technology, Barcelona, Spain
D. Bogavac, M. Bosman, M. P. Casado, L. Castillo Garcia, F. A. Förster, G. Giannini, E. L. Gkougkousis, J. Glatzer, S. Gonzalez Fernandez, C. Grieco, S. Grinstein, A. Juste Rozas, S. Kazakos, I. Korolkov, M. Martinez, L. M. Mir, C. Moreno Martinez, J. L. Munoz Martinez, N. Orlando, A. Pacheco Pages, C. Padilla Aranda, I. Riu, R. Rosten, A. Salvador Salas, A. Sonay, S. Terzo, T. R. Van Daalen & D. Vazquez Furelos
Institute of High Energy Physics, Chinese Academy of Sciences, Bei**g, China
M. K. Ayoub, J. Barreiro Guimarães da Costa, H. J. Cheng, X. Chu, H. Cui, Y. Fang, Y. Fang, J. A. García Pascual, Y. F. Hu, Y. Huang, M. G. Kurth, M. Li, Z. Liang, P. Liu, Y. Liu, X. Lou, F. Lyu, Q. Ouyang, K. Ran, L. Y. Shan, D. Xu, K. Zhang, Y. Zhang, C. Zhu, H. Zhu & X. Zhuang
Physics Department, Tsinghua University, Bei**g, China
X. Chen, W. Ding, B. Li, Y. Xu, D. F. Zhang & G. Zhang
Department of Physics, Nan**g University, Nan**g, China
H. Chen, S. J. Chen, A. De Maria, A. D’onofrio, L. Han, X. Huang, Z. Jia, S. **, F. L. Lucio Alves, W. Wang, H. Ye, B. Zhang & L. Zhang
University of Chinese Academy of Science (UCAS), Bei**g, China
X. Chu, H. Cui, Y. F. Hu, M. G. Kurth, M. Li, Y. Liu, K. Ran, Y. Zhang & C. Zhu
Institute of Physics, University of Belgrade, Belgrade, Serbia
E. Bakos, Dj. Sijacki, N. Vranjes, M. Vranjes Milosavljevic & L. Živković
Department for Physics and Technology, University of Bergen, Bergen, Norway
T. Buanes, J. I. Djuvsland, G. Eigen, N. Fomin, G. R. Lee, A. Lipniacka, B. Martin dit Latour, B. Stugu & A. Traeet
Physics Division, Lawrence Berkeley National Laboratory and University of California, Berkeley, CA, USA
X. Ai, D. J. A. Antrim, R. M. Barnett, J. Beringer, P. Calafiura, F. Cerutti, A. Ciocio, J. Dickinson, A. Dimitrievska, E. M. Duffield, K. Einsweiler, M. Garcia-Sciveres, C. Gonzalez Renteria, H. M. Gray, C. Haber, S. Han, T. Heim, I. Hinchliffe, X. Ju, K. Krizka, C. Leggett, Z. Marshall, W. P. McCormack, J. A. Mcfayden, M. Muškinja, B. P. Nachman, G. J. Ottino, S. Pagan Griso, V. R. Pascuzzi, E. Pianori, E. D. Resseguie, S. N. Santpur, M. Shapiro, V. Tsulaia, H. Wang, H. T. Yang & W-M. Yao
Institut für Physik, Humboldt Universität zu Berlin, Berlin, Germany
N. B. Atlay, A. Bandyopadhyay, D. Berge, D. Biedermann, J. Dietrich, S. Grancagnolo, C. Issever, K. Kreul, H. Lacker, T. Lohse, S. Mergelmeyer, M. Michetti, Y. S. Ng, C. Scharf, F. Schenck, P. Seema & T. Theveneaux-Pelzer
Albert Einstein Center for Fundamental Physics and Laboratory for High Energy Physics, University of Bern, Bern, Switzerland
J. K. Anders, H. P. Beck, M. Chatterjee, A. Ereditato, A. Fehr, L. Franconi, S. Haug, N. Lehmann, A. Miucci, M. S. Weber & T. D. Weston
School of Physics and Astronomy, University of Birmingham, Birmingham, UK
P. P. Allport, J. Bracinik, D. L. Briglin, D. G. Charlton, A. S. Chisholm, T. Fitschen, P. M. Freeman, L. Gonella, F. Gonnella, N. A. Gorasia, C. M. Hawkes, S. J. Hillier, J. J. Kempster, J. Kendrick, D. J. Lewis, J. H. Lindon, A. M. Mendes Jacques Da Costa, T. J. Neep, P. R. Newman, K. Nikolopoulos, E. Reynolds, C. J. Simpson-allsop, A. Stampekis, J. P. Thomas, P. D. Thompson, R. J. Turner, R. A. Vallance, G. S. Virdee, R. J. Ward, A. T. Watson & M. F. Watson
Facultad de Ciencias y Centro de Investigaciónes, Universidad Antonio Nariño, Bogotá, Colombia
D. Moreno & G. Navarro
Departamento de Física, Universidad Nacional de Colombia, Bogotá, Colombia
C. Sandoval
Dipartimento di Fisica, INFN Bologna and Universita’ di Bologna, Bologna, Italy
G. L. Alberghi, F. Alfonsi, S. Biondi, G. Cabras, G. Carratta, A. Cervelli, L. Clissa, S. De Castro, L. Fabbri, M. Franchini, A. Gabrielli, F. Lasagni Manghi, L. Massa, M. Romano, N. Semprini-Cesari, A. Sidoti, M. Sioli, K. Todome, S. Valentinetti, M. Villa, C. Vittori & A. Zoccoli
INFN Sezione di Bologna, Bologna, Italy
G. L. Alberghi, F. Alfonsi, L. Bellagamba, S. Biondi, D. Boscherini, A. Bruni, G. Bruni, M. Bruschi, G. Cabras, G. Carratta, A. Cervelli, L. Clissa, S. De Castro, L. Fabbri, M. Franchini, A. Gabrielli, B. Giacobbe, F. Lasagni Manghi, L. Massa, M. Negrini, A. Polini, L. Rinaldi, M. Romano, C. Sbarra, N. Semprini-Cesari, A. Sidoti, M. Sioli, K. Todome, S. Valentinetti, M. Villa, C. Vittori & A. Zoccoli
Physikalisches Institut, Universität Bonn, Bonn, Germany
P. Bauer, P. Bechtle, F. Beisiegel, F. U. Bernlochner, I. Brock, M. Cristinziani, K. Desch, C. Deutsch, F. G. Diaz Capriles, J. Dingfelder, P. J. Falke, C. Grefe, M. Hamer, M. C. Hansen, F. Hinterkeuser, T. Holm, M. Huebner, F. Huegging, O. Kivernyk, T. Klingl, P. T. Koenig, H. Krüger, K. Lantzsch, T. Lenz, P. Mättig, A. Melzer, R. Moles-Valls, Ö.O. Öncel, D. Pohl, L. K. Schildgen, C. Vergis, E. Von Toerne, P. Wagner & N. Wermes
Department of Physics, Boston University, Boston, MA, USA
J. M. Butler, K. D. Finelli, A. D. Sherman, Z. Yan & E. Yigitbasi
Department of Physics, Brandeis University, Waltham, MA, USA
J. R. Bensinger, L. J. Bergsten, P. Bhattarai, C. Blocker, J. Chen, D. Dodsworth, M. Goblirsch-Kolb, H. Herde, K. O’Connor, Z. M. Schillaci & G. Sciolla
Transilvania University of Brasov, Brasov, Romania
S. Popa
Horia Hulubei National Institute of Physics and Nuclear Engineering, Bucharest, Romania
C. Alexa, A. Chitan, I. A. Cioară, D. A. Ciubotaru, S. Constantinescu, M. Dobre, O. A. Ducu, A. E. Dumitriu, A. A. Geanta, A. **aru, V. S. Martoiu, J. Maurer, A. Olariu, D. Pietreanu, M. Renda, M. Rotaru, G. Stoicea, G. Tarna, A. Tudorache, V. Tudorache & M. E. Vasile
Department of Physics, Alexandru Ioan Cuza University of Iasi, Iasi, Romania
C. Agheorghiesei
Physics Department, National Institute for Research and Development of Isotopic and Molecular Technologies, Cluj-Napoca, Romania
G. A. Popeneciu
University Politehnica Bucharest, Bucharest, Romania
R. Hobincu
West University in Timisoara, Timisoara, Romania
P. M. Gravila
Faculty of Mathematics, Physics and Informatics, Comenius University, Bratislava, Slovakia
R. Astalos, D. Babal, P. Bartos, T. Blazek, M. Dubovsky, S. Hyrych, O. Majersky, M. Melo, M. Racko, I. Sykora, S. Tokár & T. Ženiš
Department of Subnuclear Physics, Institute of Experimental Physics of the Slovak Academy of Sciences, Kosice, Slovak Republic
D. Bruncko, P. Murin, J. Smiesko, F. Sopkova, P. Strizenec & J. Urban
Physics Department, Brookhaven National Laboratory, Upton, NY, USA
K. Assamagan, G. Barone, M. Begel, M. Benoit, E. Brost, V. Cavaliere, H. Chen, G. D’amen, J. Elmsheuser, H. A. Gordon, K. K. Hill, G. Iakovidis, C. W. Kalderon, A. Klimentov, V. Kouskoura, E. Lançon, F. Lanni, C. A. Lee, S. Lim, H. B. Liu, D. Lynn, H. Ma, T. Maeno, D. Matakias, J. L. Nagle, P. Nilsson, M. A. Nomura, D. Oliveira Damazio, J. Ouellette, D. V. Perepelitsa, M.-A. Pleier, V. Polychronakos, S. Protopopescu, S. Rajagopalan, G. Redlinger, J. Roloff, B. D. Seidlitz, C. Serfon, S. Snyder, P. Steinberg, S. A. Stucci, A. Tricoli, A. Undrus, C. Weber, T. Wenaus, L. Xu & S. Ye
Departamento de Física, Universidad de Buenos Aires, Buenos Aires, Argentina
M. F. Daneri, M. R. Devesa, G. Marceca, G. Otero y Garzon, R. Piegaia & M. Toscani
California State University, Long Beach, CA, USA
H. S. Bawa, Y. S. Gao, K. Grimm, J. Moss & A. J. Parker
Cavendish Laboratory, University of Cambridge, Cambridge, UK
J. R. Batley, O. Brandt, J. D. Chapman, J. W. Cowley, W. J. Fawcett, L. Henkelmann, J. C. Hill, L. B. A. H. Hommels, C. G. Lester, C. Y. Lin, C. Malone, D. L. Noel, H. A. Pacey, M. A. Parker, C. J. Potter, D. Robinson, R. Tombs, C. P. Ward & S. Williams
Department of Physics, University of Cape Town, Cape Town, South Africa
R. J. Atkin, J. M. Keaveney, C. Mwewa & S. Yacoob
Department of Mechanical Engineering Science, University of Johannesburg, Johannesburg, South Africa
D. Boye, S. H. Connell, N. Govender & L. Truong
School of Physics, University of the Witwatersrand, Johannesburg, South Africa
L. D. Christopher, S. Dahbi, Y. Hernández Jiménez, H. Jivan, D. Kar, J. E. Mdhluli, B. R. Mellado Garcia, D. Roy, X. Ruan, E. M. Shrif, E. Sideras Haddad, S. Sinha, K. G. Tomiwa & S. E. von Buddenbrock
Department of Physics, Carleton University, Ottawa, ON, Canada
A. Bellerive, C. C. Chau, D. Gillberg, J. Heilman, R. F. H. Hunter, J. S. Keller, C. Klein, T. Koffas, A. Laurier, L. S. Miller, M. Naseri, F. G. Oakham, M. G. Vincter, S. A. Weber & N. Zakharchuk
Faculté des Sciences Ain Chock, Réseau Universitaire de Physique des Hautes Energies, Université Hassan II, Casablanca, Morocco
D. Benchekroun, K. Bouaouda, Z. Chadi, A. Hoummada & H. Imam
Faculté des Sciences, Université Ibn-Tofail, Kenitra, Morocco
M. Gouighri
Faculté des Sciences, Université Mohamed Premier and LPTPM, Oujda, Morocco
J. Assahsah, J. E. Derkaoui & M. Ouchrif
Faculté des sciences, Université Mohammed V, Rabat, Morocco
S. Batlamous, R. Cherkaoui El Moursli, H. El Jarrari, F. Fassi, H. Hamdaoui, B. Ngair, Y. Tayalati & M. Zaazoua
CERN, Geneva, Switzerland
A. Abed Abud, A. Ahmad, S. Alderweireldt, M. Aleksa, C. Allaire, C. Amelung, N. Aranzabal, A. J. Armbruster, G. Avolio, M-S. Barisits, R. Bielski, T. Bisanz, J. Boyd, L. Brenner, P. Butti, N. Calace, S. Camarda, C. Camincher, S. Campana, T. Carli, A. Catinaccio, A. Cattai, A. E. C. Coimbra, P. Czodrowski, F. Dachs, V. Dao, A. Dell’Acqua, P. O. Deviveiros, A. Di Girolamo, F. Dittus, O. A. Ducu, A. Dudarev, M. Dührssen, M. Dyndal, N. Ellis, M. Elsing, S. Falke, P. Farthouat, P. Fassnacht, D. Francis, D. Froidevaux, A. Gabrielli, L. Goossens, B. Gorini, S. Guindon, R. J. Hawkings, L. Heinrich, C. Helsens, A. M. Henriques Correia, L. Hervas, A. Hoecker, M. Huhtinen, P. Iengo, T. Javůrek, J. Jovicevic, M. Kiehn, P. Klimek, F. Klimpel, T. Klioutchnikova, N. M. Köhler, A. Krasznahorkay, S. Kuehn, E. S. Kuwertz, M. Lassnig, G. Lehmann Miotto, L. Longo, A. Marzin, S. Meehan, L. Meng, J. Montejo Berlingen, A. K. Morley, G. Mornacchi, L. Morvaj, P. Moschovakos, A. M. Nairz, M. Nessi, M. Nordberg, S. Palestini, T. Pauly, H. Pernegger, S. Perrella, B. A. Petersen, L. Pontecorvo, M. E. Pozo Astigarraga, M. Queitsch-Maitland, M. Raymond, C. Rembser, C. Rizzi, S. Roe, A. Rummler, M. Saimpert, A. Salzburger, J. Samarati, A. Sanchez Pineda, S. Schlenker, J. Schovancova, A. Sharma, O. Sidiropoulou, M. V. Silva Oliveira, C. A. Solans Sanchez, G. Spigo, G. A. Stewart, M. C. Stockton, H. Ten Kate, A. N. Tuna, G. Unal, T. Vafeiadis, A. Vallier, W. Vandelli, T. Vazquez Schroeder, R. Vuillermet, P. S. Wells, T. Wengler, S. Wenig, H. G. Wilkens, C. J. S. Young, S. Zambito, D. Zanzi & L. Zwalinski
Enrico Fermi Institute, University of Chicago, Chicago, IL, USA
R. W. Gardner, M. D. Hank, K. Hildebrand, T. R. Holmes, L. A. Horyn, Y. K. Kim, J. K. K. Liu, D. W. Miller, M. J. Oreglia, J. E. Pilcher, D. Schaefer, T. Seiss, M. J. Shochet, E. A. Smith, I. Vukotic & R. Zou
LPC, Université Clermont Auvergne, CNRS/IN2P3, Clermont-Ferrand, France
M. N. Agaras, W. M. Barbe, D. Boumediene, D. Calvet, S. Calvet, J. Donini, F. A. Jimenez Morales, R. Madar, T. Megy, E. Nibigira, L. Rustige, C. Santoni & F. Vazeille
Nevis Laboratory, Columbia University, Irvington, NY, USA
A. Angerami, G. Brooijmans, J. Chen, B. Cole, A. Emerman, B. J. Gilbert, J. L. Gonski, Q. Hu, A. Kahn, K. E. Kennedy, D. J. Mahon, S. Mohapatra, J. A. Parsons, P. M. Tuts, D. M. Williams & P. Yin
Niels Bohr Institute, University of Copenhagen, Copenhagen, Denmark
O. S. AbouZeid, A. Camplani, M. Dam, J. B. Hansen, J. D. Hansen, P. H. Hansen, R. Ignazzi, D. S. Nielsen, T. C. Petersen, C. Wiglesworth & S. Xella
Dipartimento di Fisica, Università della Calabria, Rende, Italy
M. Capua, G. Carducci, G. Crosetti, D. Malito, A. Mastroberardino, E. Meoni, D. Salvatore, M. Schioppa & E. Tassi
INFN Gruppo Collegato di Cosenza, Laboratori Nazionali di Frascati, Frascati, Italy
M. Capua, G. Carducci, G. Crosetti, I. Gnesi, D. Malito, A. Mastroberardino, E. Meoni, D. Salvatore, M. Schioppa & E. Tassi
Physics Department, Southern Methodist University, Dallas, TX, USA
A. Betti, A. M. Deiana, K. J. C. Leney, M. A. McKay, C. D. Milke, R. Narayan, S. Parajuli, S. J. Sekula, R. Stroynowski, P. Wang & J. Ye
Physics Department, University of Texas at Dallas, Richardson, TX, USA
S. W. Ferguson, J. M. Izen, B. Meirose & K. Reeves
National Centre for Scientific Research “Demokritos”, Agia Paraskevi, Greece
G. Fanourakis, T. Geralis, M. M. Prapa & G. Stavropoulos
Department of Physics, Stockholm University, Stockholm, Sweden
S. Y. Andrean, F. Backman, L. Barranco Navarro, C. Bohm, C. Clement, S. Hellman, A. Kastanas, X. Lou, D. A. Milstead, T. Moa, M. E. Nelson, P. Pasuwan, L. Pereira Sanchez, N. W. Shaikh, S. B. Silverstein, J. Sjölin, S. Strandberg, A. Strubig, E. Valdes Santurio & V. Wallangen
Oskar Klein Centre, Stockholm, Sweden
S. Y. Andrean, F. Backman, L. Barranco Navarro, C. Clement, S. Hellman, A. Kastanas, X. Lou, D. A. Milstead, T. Moa, M. E. Nelson, P. Pasuwan, L. Pereira Sanchez, N. W. Shaikh, J. Sjölin, S. Strandberg, A. Strubig, E. Valdes Santurio & V. Wallangen
Deutsches Elektronen-Synchrotron DESY, Hamburg and Zeuthen, Germany
S. Amoroso, L. Aperio Bella, J.-H. Arling, A. Basalaev, C. Becot, J. K. Behr, I. Bloch, F. Braren, K. Brendlinger, B. Brüers, J. T. P. Burr, S. Carrá, Y-H. Chen, T. Daubney, Y. Delabat Diaz, S. Díez Cornell, B. Dutta, J. Ferrando, G. Gaycken, N. E. K. Gillwald, P. C. F. Glaysher, A. Glazov, I. M. Gregor, K. Grevtsov, A. Guida, R. Gupta, S. Heim, B. Heinemann, L. Helary, K. H. Hiller, C. Issever, R. M. Jacobs, N. Jeong, J. Katzy, V. Kitali, D. Kuechler, J. T. Kuechler, T. Kuhl, W. A. Leight, X. Li, Y. Li, M. Liberatore, A. Linss, Y. Liu, E. M. Lobodzinska, F. Meloni, P. Moder, K. Mönig, R. F. Naranjo Garcia, T. Naumann, F. Nechansky, P. Pani, K. Peters, C. S. Pollard, K. Potamianos, G. Pownall, D. M. Rauch, A. Renardi, S. Richter, O. Rifki, M. Rimoldi, P. Rivadeneira, M. Robin, E. Rossi, L. Rossini, J. A. Sabater Iglesias, C. O. Sander, S. Schmitt, C. Seitz, D. South, M. M. Stanitzki, M. Stegler, N. A. Styles, K. Tackmann, E. A. Thompson, F. Tsai, L. Valéry, J. Von Ahnen, C. Wanotayaroj, A. F. Wongel, S. D. Worm & Y. C. Yap
Lehrstuhl für Experimentelle Physik IV, Technische Universität Dortmund, Dortmund, Germany
T. Dado, S. Dungs, J. Erdmann, E. M. Freundlich, G. Gessner, K. Kroeninger, T. Kupfer, O. Nackenhorst, I. Nitsche, L. Rustige, K. Sedlaczek, J. Weingarten, B. Wendland & S. V. Zeißner
Institut für Kern- und Teilchenphysik, Technische Universität Dresden, Dresden, Germany
A. Berthold, C. Bittrich, D. Duschinger, T. Herrmann, M. Hils, P. Horn, F. Iltzsche, D. Kirchmeier, M. Kobel, W. F. Mader, N. Madysa, M. Maerker, J. Manjarres Ramos, S. Morgenstern, A.Nag Nag, O. Novgorodova, F. Siegert, A. Straessner, S. Todt, H. Torres & C. Wiel
Department of Physics, Duke University, Durham, NC, USA
A. T. H. Arce, J. B. Beacham, D. R. Davis, M. G. Eggleston, M. B. Epland, M. Feng, A. T. Goshaw, A. Kotwal, M. C. Kruse, K. Pachal, S. Sen & P. Zhao
SUPA, School of Physics and Astronomy, University of Edinburgh, Edinburgh, UK
T. M. Carter, P. J. Clark, S. M. Farrington, M. Faucci Giannelli, Y. Gao, G. N. Hamity, A. Hasib, M. P. Heath, C. Leonidopoulos, V. J. Martin, L. Mijović, S. Palazzo, A. Søgaard, E. P. Takeva, A. J. Taylor, N. Themistokleous, E. M. Villhauer, A. Vishwakarma & B. M. Wynne
INFN e Laboratori Nazionali di Frascati, Frascati, Italy
M. Antonelli, C. Arcangeletti, M. Beretta, V. Chiarella, G. Maccarrone, G. Mancini, A. Sansoni, M. Testa & E. Vilucchi
Physikalisches Institut, Albert-Ludwigs-Universität Freiburg, Freiburg, Germany
S. Argyropoulos, F. Becherer, C. Garcia-Argos, S. Gargiulo, M. Guth, C. Heidegger, K. K. Heidegger, G. Herten, D. Hohn, J. C. Honig, K. Jakobs, P. Jenni, S. Jiggins, T. Klapdor-Kleingrothaus, A. Knue, K. Köneke, O. Kuprash, U. Landgraf, V. S. Lang, A. Lösle, V. Magerl, U. Parzefall, V. Plesanovs, A. Rodriguez Rodriguez, B. Roland, B. Rottler, F. Rühr, Z. Rurikova, D. Sammel, F. Sauerburger, K. E. Schleicher, P. G. Scholer, M. Schumacher, D. Sperlich, C. Weiser, L. A. M. Wiik-Fuchs, B. T. Winter, J. Wollrath & S. Zimmermann
II. Physikalisches Institut, Georg-August-Universität Göttingen, Göttingen, Germany
K. Abeling, B. Achkar, H. C. Beck, M. Bindi, P. Bokan, E. Buschmann, T. Dreyer, L. O. Gerlach, R. Goncalves Gama, J. Grosse-Knetter, M. Janus, G. Kawamura, A. Kirchhoff, S. Lai, J. C. Lange, J. D. Mellenthin, S. Mobius, M. Niemeyer, S. Oerdek, T. Peiffer, I. Pokharel, A. Quadt, E. Shabalina, A. Skaf, F. Sohns, J. Veatch & K. Zoch
Département de Physique Nucléaire et Corpusculaire, Université de Genève, Geneva, Switzerland
S. Adorni, E. Akilli, C. S. Amrouche, C. Antel, A. Clark, D. Della Volpe, A. Dubreuil, D. Ferrere, S. Gadatsch, T. Golling, S. Gonzalez-Sevilla, G. Iacobucci, T. Iizawa, R. Jansky, A. L. Lionti, P. Mermod, H. R. Nindhito, C. E. Pandini, L. Paolozzi, R. Poggi, J. A. Raine, D. Salamani, S. Schramm, A. Sfyrla, D. M. S. Sultan, X. Wu & E. Zaffaroni
Dipartimento di Fisica, Università di Genova, Genoa, Italy
D. Barberis, G. Gagliardi, A. Gaudiello, A. Lapertosa, F. Parodi, M. Sannino, C. Schiavi, F. Sforza & C. Varni
INFN Sezione di Genova, Genoa, Italy
D. Barberis, A. Coccaro, G. Darbo, G. Gagliardi, A. Gaudiello, C. Gemme, A. Lapertosa, P. Morettini, F. Parodi, S. Passaggio, L. P. Rossi, M. Sannino, C. Schiavi, F. Sforza & C. Varni
II. Physikalisches Institut, Justus-Liebig-Universität Giessen, Giessen, Germany
D. Caforio, M. Düren & H. Stenzel
SUPA, School of Physics and Astronomy, University of Glasgow, Glasgow, UK
R. L. Bates, A. Blue, A. G. Borbély, W. D. Breaden Madden, D. Britton, A. G. Buckley, P. J. Bussey, C. M. Buttar, G. Callea, I. A. Connelly, W. R. Cunningham, A. T. Doyle, F. Fabbri, C. Gray, J. Howarth, J. Jamieson, L. Mince, V. O’Shea, M. Owen, B. Ravina, A. G. Rennie, A. Robson, D. P. Spiteri, N. Warrack & K. Wraight
LPSC, Université Grenoble Alpes, CNRS/IN2P3, Grenoble INP, Grenoble, France
J. Collot, S. Crépé-Renaudin, P. A. Delsart, M. H. Genest, R. Hulsken, M. Kuna, F. Ledroit-Guillon, A. Lleres, A. Lucotte, F. Malek, D. M. Portillo Quintero, J. Stark, B. Trocmé & X. Yang
Laboratory for Particle Physics and Cosmology, Harvard University, Cambridge, MA, USA
N. Asbah, B. A. Bullard, K. F. Di Petrillo, M. Franklin, J. Huth, L. Lee, M. Morii, G. Rabanal Bolanos & A. M. Wang
Department of Modern Physics and State Key Laboratory of Particle Detection and Electronics, University of Science and Technology of China, Hefei, China
Z. Barnovska-Blenessy, A. Baroncelli, C. Chen, J. Chen, Y. Chen, J. Gao, Y. Guo, K. Han, L. Han, F. He, Y. Huang, Y. Jiang, D. Krasnopevtsev, H. Li, Q. Y. Li, J. B. Liu, M. Liu, M. Y. Liu, X. Liu, Y. W. Liu, M. Lu, R. Ospanov, H. Peng, M. Sessa, X. Su, C. Wang, R. Wang, W. T. Wang, W. X. Wang, Y. Wang, Y. Wu, X. **e, H. Xu, H. Xu, S. Yang, X. Yang, Z. Yang, L. Zhang, Z. Zhao, H. L. Zhu & Y. Zhu
Institute of Frontier and Interdisciplinary Science and Key Laboratory of Particle Physics and Particle Irradiation (MOE), Shandong University, Qingdao, China
M. J. Da Cunha Sargedas De Sousa, D. Du, C. Feng, H. Li, Z. Li, L. L. Ma, K. Tariq, Z. Xu, X. Yang, R. Yuan, X. Zhang & C. G. Zhu
School of Physics and Astronomy, Shanghai Jiao Tong University, KLPPAC-MoE, SKLPPC, Shanghai, China
N. Brahimi, B. Dong, J. Guo, S. Hu, C-Q. Li, F. Li, J. Li, L. Li, S. Li, K. Liu, M. Mittal, N. Nishu, D. Sampsonidou, W. Su, C. Wang, J. Yan, H. J. Yang, X. Zhang & N. Zhou
Tsung-Dao Lee Institute, Shanghai, China
N. Brahimi, Y. Duan, C. Kato, C-Q. Li, S. Li, K. Liu, D. Sampsonidou, W. Su & H. J. Yang
Kirchhoff-Institut für Physik, Ruprecht-Karls-Universität Heidelberg, Heidelberg, Germany
V. Andrei, F. Bartels, M. Dunford, S. Franchino, M. Klassen, T. Mkrtchyan, F. Napolitano, P. S. Ott, D. F. Rassloff, H-C. Schultz-Coulon, T. M. Spieker, R. Stamen, P. Starovoitov, S. M. Weber, M. Wessels & X. Yue
Physikalisches Institut, Ruprecht-Karls-Universität Heidelberg, Heidelberg, Germany
A. E. Bolz, M. M. Czurylo, S. J. Dittmeier, D. E. Ferreira de Lima, A. Krishnan, A. Schoening & L. Vigani
Faculty of Applied Information Science, Hiroshima Institute of Technology, Hiroshima, Japan
Y. Nagasaka
Department of Physics, Chinese University of Hong Kong, Shatin, N.T., Hong Kong, China
H. C. Cheng, M. C. Chu, L. R. Flores Castillo, J. M. Iturbe Ponce, T. S. Lau, A. Salvucci, J. Wang & Z. Zhang
Department of Physics, University of Hong Kong, Hong Kong, China
C. Y. Lo, D. Paredes Hernandez, C. Peng, K. C. Tam & Y. Tu
Department of Physics and Institute for Advanced Study, Hong Kong University of Science and Technology, Clear Water Bay, Kowloon, Hong Kong, China
K. Lie, K. Prokofiev, J. **ang & T. Yang
Department of Physics, National Tsing Hua University, Hsinchu, Taiwan
K. Cheung, P. J. Hsu & Y. J. Lu
IJCLab, Université Paris-Saclay, CNRS/IN2P3, 91405, Orsay, France
C. Agapopoulou, K. Al Khoury, H. Atmani, A. Bassalat, J. B. De Vivie De Regie, D. Delgove, C. Delporte, L. Duflot, M. Escalier, L. Fayard, D. Fournier, A. Ghosh, J.-F. Grivaz, A. Guerguichon, D. Hohov, J. Hrivnac, L. Iconomidou-Fayard, A. Kotsokechagia, A. Lounis, N. Makovec, N. Morange, M. M. Perego, P. Puzo, D. Rousseau, G. Rybkin, S. Sacerdoti, A. C. Schaffer, L. Serin, S. Simion, R. Tanaka, A. Trofymov, D. Varouchas, D. Zerwas & Z. Zhang
Department of Physics, Indiana University, Bloomington, IN, USA
P. Calfayan, H. Evans, B. C. Forland, C. A. Johnson, R. Kopeliansky, S. Lammers, R. A. Linck, F. Luehring, C. Meyer, G. Myers, G. Palacino, C. P. A. Roland & D. Zieminska
INFN Gruppo Collegato di Udine, Sezione di Trieste, Udine, Italy
B. S. Acharya, M. Cobal, M. Faraj, M. P. Giordani, G. Giugliarelli, J. Magro, G. Panizzo, M. Pinamonti, A. Sanchez Pineda, A. Sbrizzi, L. Serkin & R. Soualah
ICTP, Trieste, Italy
B. S. Acharya & L. Serkin
Dipartimento Politecnico di Ingegneria e Architettura, Università di Udine, Udine, Italy
M. Cobal, M. Faraj, M. P. Giordani, G. Giugliarelli, J. Magro, G. Panizzo, M. Pinamonti, A. Sanchez Pineda, A. Sbrizzi & R. Soualah
INFN Sezione di Lecce, Lecce, Italy
G. Chiodini, E. Gorini, F. G. Gravili, M. Primavera, M. Reale, E. J. Schioppa, S. Spagnolo & A. Ventura
Dipartimento di Matematica e Fisica, Università del Salento, Lecce, Italy
E. Gorini, F. G. Gravili, M. Reale, E. J. Schioppa, S. Spagnolo & A. Ventura
INFN Sezione di Milano, Milan, Italy
G. Alimonti, A. Andreazza, L. Carminati, M. Citterio, S. D’Auria, M. Fanti, D. Giugni, T. Lari, M. Lazzaroni, C. Meroni, S. Monzani, D. P. Mungo, A. Murrone, L. Perini, F. Ragusa, S. Resconi, A. Stabile, G. F. Tartarelli, C. Troncon & R. Turra
Dipartimento di Fisica, Università di Milano, Milan, Italy
A. Andreazza, L. Carminati, S. D’Auria, M. Fanti, M. Lazzaroni, D. P. Mungo, A. Murrone, L. Perini, F. Ragusa & A. Stabile
INFN Sezione di Napoli, Naples, Italy
A. Aloisio, M. G. Alviggi, V. Canale, G. Carlino, F. Cirotto, F. Conventi, R. De Asmundis, M. Della Pietra, C. Di Donato, A. Doria, A. Giannini, V. Izzo, M. Lavorgna, P. Massarotti, L. Merola, E. Rossi & G. Sekhniaidze
Dipartimento di Fisica, Università di Napoli, Naples, Italy
A. Aloisio, M. G. Alviggi, V. Canale, F. Cirotto, M. Della Pietra, C. Di Donato, A. Giannini, M. Lavorgna, P. Massarotti, L. Merola & E. Rossi
INFN Sezione di Pavia, Pavia, Italy
E. M. Farina, R. Ferrari, G. Gaudio, G. Introzzi, A. Kourkoumeli-Charalampidi, A. Lanza, M. Livan, A. Negri, L. Pezzotti, G. Polesello, D. M. Rebuzzi, A. Rimoldi, G. Rovelli, S. Sottocornola & V. Vercesi
Dipartimento di Fisica, Università di Pavia, Pavia, Italy
E. M. Farina, G. Introzzi, A. Kourkoumeli-Charalampidi, M. Livan, A. Negri, L. Pezzotti, D. M. Rebuzzi, A. Rimoldi, G. Rovelli & S. Sottocornola
INFN Sezione di Pisa, Pisa, Italy
A. Annovi, N. V. Biesuz, M. Calvetti, V. Cavasinni, G. Chiarelli, G. Di Gregorio, P. Francavilla, P. Giannetti, S. Leone, P. Mastrandrea, C. Roda, F. Scuri & M. Verducci
Dipartimento di Fisica E. Fermi, Università di Pisa, Pisa, Italy
N. V. Biesuz, M. Calvetti, V. Cavasinni, G. Di Gregorio, P. Francavilla, P. Mastrandrea, C. Roda & M. Verducci
INFN Sezione di Roma, Rome, Italy
F. Anulli, P. Bagnaia, M. Bauce, C. Bini, N. Bruscino, A. R. Chomont, M. Corradi, D. De Pedis, A. De Salvo, F. A. Di Bello, S. Falciano, S. Francescato, G. Frattari, P. Gauzzi, S. Gentile, S. Giagu, V. Ippolito, M. Kado, F. Lacava, I. Longarini, C. Luci, L. Luminari, A. Nisati, E. Pasqualucci, A. Policicchio, S. Rosati, L. Sabetta, F. Safai Tehrani, D. Vannicola, R. Vari & S. Veneziano
Dipartimento di Fisica, Sapienza Università di Roma, Rome, Italy
P. Bagnaia, M. Bauce, C. Bini, N. Bruscino, A. R. Chomont, M. Corradi, F. A. Di Bello, S. Francescato, G. Frattari, P. Gauzzi, S. Gentile, S. Giagu, V. Ippolito, M. Kado, F. Lacava, I. Longarini, C. Luci, A. Policicchio, L. Sabetta & D. Vannicola
INFN Sezione di Roma Tor Vergata, Rome, Italy
G. Aielli, E. Alunno Camelia, A. Caltabiano, P. Camarri, R. Cardarelli, L. Cerrito, U. De Sanctis, M. De Santis, L. Dell’Asta, A. Di Ciaccio, F. Giuli, B. Liberti, S. Loffredo, L. Marcoccia, L. Pizzimento, A. Rocchi & M. Vanadia
Dipartimento di Fisica, Università di Roma Tor Vergata, Rome, Italy
G. Aielli, E. Alunno Camelia, A. Caltabiano, P. Camarri, L. Cerrito, U. De Sanctis, M. De Santis, L. Dell’Asta, A. Di Ciaccio, F. Giuli, S. Loffredo, L. Marcoccia, L. Pizzimento, A. Rocchi & M. Vanadia
INFN Sezione di Roma Tre, Rome, Italy
M. Biglietti, M. T. Camerlingo, V. D’Amico, B. Di Micco, R. Di Nardo, A. Farilla, M. Iodice, L. Martinelli, D. Orestano, F. Petrucci, E. Rossi & G. Salamanna
Dipartimento di Matematica e Fisica, Università Roma Tre, Rome, Italy
M. T. Camerlingo, V. D’Amico, B. Di Micco, R. Di Nardo, L. Martinelli, D. Orestano, F. Petrucci, E. Rossi & G. Salamanna
INFN-TIFPA, Trento, Italy
A. Di Luca, F. M. Follega, G. T. Forcolin, R. Iuppa & E. Ricci
Università degli Studi di Trento, Trento, Italy
A. Di Luca, F. M. Follega, G. T. Forcolin, R. Iuppa & E. Ricci
Institut für Astro- und Teilchenphysik, Leopold-Franzens-Universität, Innsbruck, Austria
J. Guenther, D. Guest, T. J. Khoo, E. Kneringer & A. Manousos
University of Iowa, Iowa City, IA, USA
M. Cano Bret, A. Ghosh & U. Mallik
Department of Physics and Astronomy, Iowa State University, Ames, IA, USA
F. An, B. Chen, C. H. Chen, J. Cochran, W. D. Heidorn, H. Jiang, S. Kang, N. Krumnack, A. Lebedev, S. Lee, B. Liu, N. Magini, S. Prell, C. M. Vergel Infante, K. Yoshihara & J. Yu
Joint Institute for Nuclear Research, Dubna, Russia
F. Ahmadov, I. N. Aleksandrov, V. A. Bednyakov, I. R. Boyko, I. A. Budagov, G. A. Chelkov, A. Cheplakov, M. V. Chizhov, D. V. Dedovich, M. Demichev, A. Gongadze, M. I. Gostkin, N. Huseynov, N. Javadov, S. N. Karpov, Z. M. Karpova, E. Khramov, U. Kruchonak, V. Kukhtin, E. Ladygin, V. Lyubushkin, T. Lyubushkina, S. Malyukov, M. Mineev, E. Plotnikova, I. N. Potrap, F. Prokoshin, N. A. Rusakovich, R. Sadykov, A. Sapronov, M. Shiyakova, A. Soloshenko, S. Turchikhin, I. Yeletskikh, A. Zhemchugov & N. I. Zimine
Departamento de Engenharia Elétrica, Universidade Federal de Juiz de Fora (UFJF), Juiz de Fora, Brazil
V. Araujo Ferraz, A. S. Cerqueira, L. Manhaes de Andrade Filho & B. S. Peralva
Universidade Federal do Rio De Janeiro COPPE/EE/IF, Rio de Janeiro, Brazil
Y. Amaral Coutinho, R. Araujo Pereira, M. Begalli, L. P. Caloba, J. V. Da Fonseca Pinto, W. S. Freund, P. Gaspar, C. Maidantchik & J. M. Seixas
Instituto de Física, Universidade de São Paulo, São Paulo, Brazil
M. Donadelli & M. A. L. Leite
KEK, High Energy Accelerator Research Organization, Tsukuba, Japan
M. Aoki, K. Hanagaki, S. Higashino, Y. Ikegami, M. Ikeno, J. Kanzaki, T. Kondo, Y. Makida, A. Mizukami, K. Nagano, K. Nakamura, S. Odaka, O. Sasaki, S. Suzuki, Y. Takubo, K. Tokushuku, M. Tomoto, S. Tsuno, Y. Unno & A. Yamamoto
Graduate School of Science, Kobe University, Kobe, Japan
H. Hibi, S. Kido, H. Kurashige, J. Maeda, A. Ochi, K. Takeda & Y. Yamazaki
AGH University of Science and Technology, Faculty of Physics and Applied Computer Science, Kraków, Poland
L. Adamczyk, T. Bold, W. Dabrowski, I. Grabowska-Bold, K. W. Janas, P. A. Janus, S. Koperny, I. K. Lakomiec, K. Maj, B. Mindur, A. Ogrodnik, P. Palni & M. Przybycien
Marian Smoluchowski Institute of Physics, Jagiellonian University, Kraków, Poland
D. T. Gil, M. Palka & E. Richter-Was
Institute of Nuclear Physics Polish Academy of Sciences, Krakow, Poland
M. Bahmani, E. Banas, P. A. Bruckman de Renstrom, J. J. Chwastowski, K. M. Ciesla, S. Czekierda, D. Derendarz, B. S. Dziedzic, P. A. Erland, A. Kaczmarska, K. Korcyl, J. A. Krzysiak, Pa. Malecki, A. Olszewski, J. Olszowska, M. Slawinska, A. Smykiewicz, E. Stanecka, R. Staszewski, M. Trzebinski, A. Trzupek, M. W. Wolter, B. K. Wosiek, K. W. Woźniak & B. Zabinski
Faculty of Science, Kyoto University, Kyoto, Japan
S. Akatsuka, Y. Mino, Y. Noguchi, Y. Okazaki & T. Sumida
Kyoto University of Education, Kyoto, Japan
R. Takashima
Research Center for Advanced Particle Physics and Department of Physics, Kyushu University, Fukuoka, Japan
K. Kawagoe, D. Kobayashi, S. Oda, H. Otono, J. Tojo & N. Yamaguchi
Instituto de Física La Plata, Universidad Nacional de La Plata and CONICET, La Plata, Argentina
F. Alonso, M. T. Dova, J. Hoya, F. Monticelli, G. E. Orellana & H. Wahlberg
Physics Department, Lancaster University, Lancaster, UK
A. E. Barton, I. A. Bertram, G. Borissov, E. V. Bouhova-Thacker, H. Fox, R. C. W. Henderson, R. W. L. Jones, V. Kartvelishvili, R. E. Long, P. A. Love, D. Muenstermann, I. G. Sanderswood, M. Smizanska, A. S. Tee, A. M. Wharton, B. W. Whitmore & M. R. Yexley
Oliver Lodge Laboratory, University of Liverpool, Liverpool, UK
H. M. Borecka-Bielska, S. Burdin, W. Y. Chan, P. Dervan, M. D’Onofrio, R. Gonzalez Lopez, E. C. Graham, C. B. Gwilliam, H. S. Hayward, A. E. Jaspan, T. J. Jones, M. Klein, U. Klein, J. Kretzschmar, Z. Li, H. Lyons, A. Mehta, M. W. O’Keefe, J. Patton, N. Rompotis, C. D. Sebastiani, M. J. Sullivan, H. Teagle & J. H. Vossebeld
Department of Experimental Particle Physics, Jožef Stefan Institute and Department of Physics, University of Ljubljana, Ljubljana, Slovenia
V. Cindro, A. Filipčič, A. Gorišek, B. Hiti, B. P. Kerševan, G. Kramberger, I. Mandić, B. Maček, M. Mikuž, J. Novak & T. Novak
School of Physics and Astronomy, Queen Mary University of London, London, UK
A. J. Bevan, U. Blumenschein, M. Bona, T. P. Charman, A. A. Elliot, A. N. Fray, R. Gamboa Goni, J. M. Hays, N. D. Hehir, M. P. J. Landon, S. L. Lloyd, J. P. Mandalia, P. S. Miyagawa, A. Ramirez Morales, E. Rizvi & E. J. Thorpe
Department of Physics, Royal Holloway University of London, Egham, UK
D. K. Abhayasinghe, T. Berry, V. Boisvert, C. D. Booth, A. J. Bozson, G. Cowan, S. George, S. M. Gibson, S. D. Lawlor, H. P. Lefebvre, J. G. Panduro Vazquez, F. Pastore, J. D. Shinner, F. Spanò, P. Teixeira-Dias, D. W. Thomas, P. J. Verschuuren, S. D. Walker & L. J. Wilkins
Department of Physics and Astronomy, University College London, London, UK
G. Barbour, A. S. Bell, L. S. Borgna, J. M. Butterworth, M. Campanelli, L. D. Corpe, Z. J. Grout, C. Gutschow, G. G. Hesketh, D. P. Huang, N. Kimura, V. Konstantinides, N. Konstantinidis, A. Korn, A. C. A. Lee, Y. Ma, A. C. Martyniuk, M. Montella, A. P. Morris, E. Nurse, C. Pitman Donaldson, S. Rettie, T. Scanlon, P. Sherwood, L. Shi, A. L. Sopio, A. Vaidya, S. Van Stroud, B. M. Waugh & D. P. Yallup
Louisiana Tech University, Ruston, LA, USA
L. Sawyer & M. Wobisch
Fysiska institutionen, Lunds universitet, Lund, Sweden
T. P. A. Åkesson, S. S. Bocchetta, E. E. Corrigan, C. Doglioni, J. Geisen, K. Gregersen, E. Hansen, V. Hedberg, G. Jarlskog, E. Kellermann, B. Konya, E. Lytken, K. H. Mankinen, C. Marcon, J. U. Mjörnmark, G. A. Mullier, R. Poettgen, T. Poulsen, E. Skorda & O. Smirnova
Centre de Calcul de l’Institut National de Physique Nucléaire et de Physique des Particules (IN2P3), Villeurbanne, France
G. Rahal
Departamento de Física Teorica C-15 and CIAFF, Universidad Autónoma de Madrid, Madrid, Spain
M. Alvarez Estevez, F. Barreiro, S. Calvente Lopez, D. Camarero Munoz, J. Del Peso, C. Glasman & J. Terron
Institut für Physik, Universität Mainz, Mainz, Germany
L. Adam, M. Akbiyik, J. Balz, A. Basan, P. Berta, K. Bierwagen, B. Brickwedde, V. Büscher, J. Damp, A. C. Dudder, F. Fiedler, J. Fischer, M. Geisen, P. Gessinger-Befurt, S. Groh, R. Gugel, A. Hadef, H. Herr, K. B. Jakobi, C. Kahra, A. Kaluza, K. Kleinknecht, J. A. Kremer, A. Laudrain, L. Masetti, F. Neuhaus, S. Pataraia, S. Rave, A. Reiss, M. Robles Manzano, U. Schäfer, K. Schmieden, C. Schmitt, M. Schott, A. Schulte, R. Simoniello, A. Sydorenko, D. Ta, S. Tapprogge, E. Tzovara, R.-J. Wang, M. Weirich, N. Wieseotte & A. Wolf
School of Physics and Astronomy, University of Manchester, Manchester, UK
C. J. Birch-sykes, A. Bitadze, S. E. Clawson, J. Crane, C. Da Via, M. D’uffizi, S. Dysch, A. C. Forti, E. C. Hanson, W. Kozanecki, D. P. J. Lack, B. Le, I. Lopez Paz, J. Masik, F. J. Munoz Sanchez, A. Oh, J. R. Pater, R. F. Y. Peters, R. H. Pickles, A. D. Pilkington, D. Price, Y. Qin, J. H. Rawling, N. Scharmberg, S. M. Shaw, X. Sun, V. Vecchio, M. Vozak & T. R. Wyatt
CPPM, Aix-Marseille Université, CNRS/IN2P3, Marseille, France
G. Aad, M. Barbero, G. Bartolini, T. P. Calvet, Y. Coadou, C. Diaconu, F. Djama, A. Duperrin, L. Feligioni, E. Fortin, Z. Guo, G. D. Hallewell, F. Hubaut, E. B. F. G. Knoops, E. Le Guirriec, E. Monnier, S. Muanza, E. Nagy, H. D. N. Nguyen, E. Petit, P. Pralavorio, A. Rozanov, T. Strebler, M. Talby, S. Tisserant, J. Toth & N. K. Vu
Department of Physics, University of Massachusetts, Amherst, MA, USA
D. C. Abbott, B. Brau, J. C. Burzynski, Y. Chou, R. Coelho Lopes De Sa, C. Dallapiccola, M. Javurkova, S. Krishnamurthy, R. J. Langenberg, V. I. Martinez Outschoorn, Z. A. Meadows, E. J. W. Moyse, N. E. Pettersson, A. Picazio, G. Rosin, P. Tornambe & S. Willocq
Department of Physics, McGill University, Montreal, QC, Canada
W. S. Ahmed, A. Ambler, J. D. Bossio Sola, A. Canesse, F. Corriveau, T. Kwan, Z. Li, J. P. Mc Gowan, C. Nelson, S. H. Robertson, H. L. Russell, S. Saha, S. Stärz, B. Vachon & A. Warburton
School of Physics, University of Melbourne, Melbourne, VIC, Australia
E. L. Barberio, S. Goldfarb, E. F. McDonald, P. C. McNamara, T. Pham, F. Scutti, J. Shojaii, G. N. Taylor, F. C. Ungaro & P. Urquijo
Department of Physics, University of Michigan, Ann Arbor, MI, USA
D. Amidei, P. A. Atmasiddha, T. Dai, E. B. Diehl, C. Ferretti, P. Fleischmann, C. Geng, C. Grud, L. Guan, W. Guo, C. Hayes, M. H. Klein, D. Levin, B. Li, Y. L. Liu, S. P. Mc Kee, X. T. Meng, G. Merz, J. Qian, T. A. Schwarz, S. Sun, Z. Wang, A. S. White, X. **ao, W. Xu, S. Zhang, Z. Zheng, B. Zhou & J. Zhu
Department of Physics and Astronomy, Michigan State University, East Lansing, MI, USA
R. Brock, C. J. Buxo Vazquez, H. De la Torre, T. Farooque, W. C. Fisher, G. Halladjian, R. Hauser, D. Hayden, J. Huston, K. S. Krowpman, R. Les, K. Lin, I. Pogrebnyak, R. Schwienhorst & A. Tarek Abouelfadl Mohamed
B.I. Stepanov Institute of Physics, National Academy of Sciences of Belarus, Minsk, Belarus
S. Harkusha, Y. Kulchitsky, Y. A. Kurochkin & P. V. Tsiareshka
Research Institute for Nuclear Problems of Byelorussian State University, Minsk, Belarus
A. Hrynevich
Group of Particle Physics, University of Montreal, Montreal, QC, Canada
J-F. Arguin, G. Azuelos, G. Demontigny, B. Freund, L. G. Gagnon, D. Godin, C. Leroy, K. Mochizuki, T. Nguyen Manh & C. Papadatos
P.N. Lebedev Physical Institute of the Russian Academy of Sciences, Moscow, Russia
A. V. Akimov, F. Dubinin, I. L. Gavrilenko, R. Mashinistov, O. Meshkov, P. Y. Nechaeva, A. Shmeleva, A. A. Snesarev, V. V. Sulin, V. O. Tikhomirov & K. Zhukov
National Research Nuclear University MEPhI, Moscow, Russia
K. Belotskiy, N. L. Belyaev, O. Bulekov, A. Kurova, D. Ponomarenko, N. Proklova, D. Pyatiizbyantseva, A. Romaniouk, N. Smirnov, S. Yu. Smirnov, Y. Smirnov, E. Yu. Soldatov, S. Timoshenko & K. Vorobev
D.V. Skobeltsyn Institute of Nuclear Physics, M.V. Lomonosov Moscow State University, Moscow, Russia
L. K. Gladilin, N. Korotkova, V. A. Kramarenko, O. Meshkov, V. Sinetckii, S. Yu. Sivoklokov & L. N. Smirnova
Fakultät für Physik, Ludwig-Maximilians-Universität München, Munich, Germany
Z. P. Arrubarrena Tame, O. Biebel, G. Duckeck, F. Fischer, B. M. Flierl, D. M. Handl, N. M. Hartmann, M. G. Herrmann, R. Hertenberger, F. F. Klitzner, F. Krieter, C. E. Leitgeb, J. Lorenz, A. M. Lory, A. Mann, A. Matic, S. Mehlhase, J. Mitrevski, P. Mogg, R. S. P. Mueller, F. Rauscher, D. Schaile, E. Schanet, C. Valderanis, J. Wagner-Kuhr & R. Walker
Max-Planck-Institut für Physik (Werner-Heisenberg-Institut), Munich, Germany
T. Barillari, S. Bethke, J. Beyer, D. Britzger, D. Cieri, D. Duda, P. Gadow, F. Guescini, M. Holzbock, A. Hönle, J. Jimenez Pena, J. J. Junggeburth, A. E. Kiryunin, S. Kluth, O. Kortner, S. Kortner, D. Krauss, H. Kroha, S. R. Maschek, T. G. McCarthy, S. Menke, R. Nisius, M. B. Rendel, R. Richter, P. Rieck, R. Röhrig, M. Sahinsoy, P. Schacht, K. R. Schmidt-Sommerfeld, L. M. Scyboz, M. Spalla, S. Stonjek, A. Verbytskyi, V. M. Walbrecht & Z. Zinonos
Nagasaki Institute of Applied Science, Nagasaki, Japan
T. Fusayasu & M. Shimojima
Graduate School of Science and Kobayashi-Maskawa Institute, Nagoya University, Nagoya, Japan
H. Asada, S. Hayashida, Y. Horii, Y. Kano, T. Kawaguchi, Y. Nakahama, Y. Sano & M. Tomoto
Department of Physics and Astronomy, University of New Mexico, Albuquerque, NM, USA
A. Grummer, R. Novotny & S. C. Seidel
Institute for Mathematics, Astrophysics and Particle Physics, Radboud University/Nikhef, Nijmegen, The Netherlands
A. Aggarwal, S. Caron, N. De Groot, V. Fabiani, F. Filthaut, C. A. Gottardo, N. Ilic, A. C. König, P. Moskvitina, C. Nellist, L. Pedraza Diaz & J. F. P. Schouwenberg
Nikhef National Institute for Subatomic Physics and University of Amsterdam, Amsterdam, The Netherlands
A. Alfonsi, H. Arnold, P. J. Bakker, R. Balasubramanian, M. Bedognetti, S. Bentvelsen, G. J. Bobbink, C. D. Burgard, W. S. Chan, Y. S. Chow, A. P. Colijn, M. De Beurs, P. de Jong, F. A. Dias, T. A. du Pree, P. Ferrari, P. Kluit, S. Manzoni, A. E. McDougall, B. Moser, F. Pasquali, A. Perrevoort, A. Pizzini, H. L. Snoek, M. Stamenkovic, B. Stapf, J. J. Teoh, I. Van Vulpen, M. J. Veen, W. Verkerke, A. T. Vermeulen, J. C. Vermeulen & M. Vreeswijk
Department of Physics, Northern Illinois University, DeKalb, IL, USA
J. Adelman, T. J. Burch, D. Chakraborty, L. D’Eramo, M. Mlynarikova, R. G. Oreamuno Madriz, E. W. Parrish, P. Saha & B. L. Stamas
Budker Institute of Nuclear Physics and NSU, SB RAS, Novosibirsk, Russia
A. V. Anisenkov, E. M. Baldin, K. Beloborodov, V. S. Bobrovnikov, A. G. Bogdanchikov, A. R. Buzykaev, V. F. Kazanin, A. G. Kharlamov, T. Kharlamova, A. L. Maslennikov, D. A. Maximov, S. V. Peleganchuk, P. Podberezko, O. L. Rezanova, A. M. Soukharev, A. A. Talyshev, Yu. A. Tikhonov & V. Zhulanov
Novosibirsk State University Novosibirsk, Novosibirsk, Russia
A. V. Anisenkov, E. M. Baldin, K. Beloborodov, V. S. Bobrovnikov, A. G. Bogdanchikov, A. R. Buzykaev, V. F. Kazanin, A. G. Kharlamov, T. Kharlamova, A. L. Maslennikov, D. A. Maximov, S. V. Peleganchuk, P. Podberezko, O. L. Rezanova, A. M. Soukharev, A. A. Talyshev, Yu. A. Tikhonov & V. Zhulanov
Institute for High Energy Physics of the National Research Centre Kurchatov Institute, Protvino, Russia
A. Borisov, E. Cheremushkina, S. P. Denisov, R. M. Fakhrutdinov, A. B. Fenyuk, D. Golubkov, A. Kamenshchikov, A. N. Karyukhin, A. S. Kozhin, A. A. Minaenko, A. G. Myagkov, V. Nikolaenko, A. Ryzhov, A. A. Solodkov, O. V. Solovyanov, E. A. Starchenko, E. Tagiev, A. M. Zaitsev & O. Zenin
Institute for Theoretical and Experimental Physics named by A.I. Alikhanov of National Research Centre “Kurchatov Institute”, Moscow, Russia
A. Gavrilyuk, P. A. Gorbounov, P. B. Shatalov & I. I. Tsukerman
Department of Physics, New York University, New York, NY, USA
K. Cranmer, A. Haas, A. Held, A. I. Mincer, M. Ronzani & C. J. Treado
Ochanomizu University, Otsuka, Bunkyo-ku, Tokyo, Japan
K. Asai, M. Fujimoto & T. Kono
Ohio State University, Columbus, OH, USA
A. Boveia, S. Che, K. K. Gan, H. Kagan, C. B. Martin, B. Reynolds, S. Shrestha & E. Tolley
Homer L. Dodge Department of Physics and Astronomy, University of Oklahoma, Norman, OK, USA
B. Abbott, M. Alhroob, D. C. Frizzell, N. A. Grieser, G. Gustavino, P. Gutierrez, J. E. Lambert, M. Marjanovic, J. M. Muse, H. Severini, Y. Shen, P. Skubic, M. Strauss, J. Stupak & Q. Wang
Department of Physics, Oklahoma State University, Stillwater, OK, USA
E. Antipov, A. M. Burger, J. Cantero, J. Haley, W. Islam, A. Khanov, M. Madugoda Ralalage Don, F. Rizatdinova & E. R. Vandewall
Palacký University, RCPTM, Joint Laboratory of Optics, Olomouc, Czech Republic
K. Cerny, L. Chytka, M. Hrabovsky, T. Komarek, J. Kvita, L. Nozka & J. Pacalt
Institute for Fundamental Science, University of Oregon, Eugene, OR, USA
B. W. Allen, J. Barkeloo, J. S. Bonilla, J. E. Brau, A. Dattagupta, G. R. Gledhill, G. Gonella, J. J. Heinrich, L. Jeanty, N. A. Luongo, S. Majewski, J. Myers, I. Siral, I. M. Snyder, A. L. Steinhebel, D. M. Strom, E. Torrence, K. Whalen & F. Winklmeier
Graduate School of Science, Osaka University, Osaka, Japan
M. Hirose & H. Nanjo
Department of Physics, University of Oslo, Oslo, Norway
K. Bjørke, M. K. Bugge, D. Cameron, J. R. Catmore, V. Garonne, E. Gramstad, E. S. Haaland, A. L. Heggelund, S. Hellesund, V. Morisbak, H. Oppen, F. Ould-Saada, M. Pedersen, A. L. Read, O. Røhne, E. B. Rye, H. Sandaker & K. O. H. Vadla
Department of Physics, Oxford University, Oxford, UK
L. Ambroz, G. Artoni, W. K. Balunas, A. J. Barr, L. Beresford, D. Bortoletto, F. Celli, A. M. Cooper-Sarkar, M. G. Foti, J. A. Frost, G. E. Gallardo, E. J. Gallas, J. C. Grundy, C. Gwenlan, C. P. Hays, T. B. Huffman, K. Karava, Z. Li, L. Marchese, C. Merlassino, M. Mironova, K. Nagai, R. B. Nickerson, A. P. O’neill, S. R. Paredes Saenz, E. Schopf, I. P. J. Shipsey, H. A. Smith, B. Stanislaus, M. Stankaityte, C. Tosciri, G. H. A. Viehhauser, Y. Wei, A. R. Weidberg, P. J. Windischhofer, R. Wölker, J. Wuerzinger, G. Zemaityte & M. Zgubič
LPNHE, Sorbonne Université, Université de Paris, CNRS/IN2P3, Paris, France
T. Beau, G. Bernardi, M. Bomben, G. Calderini, R. Camacho Toro, F. Crescioli, F. Derue, R. Hankache, M. W. Krasny, D. Lacour, B. Laforge, A. Leopold, B. Malaescu, G. Marchiori, I. Nikolic-Audit, I. Nomidis, J. Ocariz, L. Pascual Dominguez, L. Poggioli, M. Ridel, L. Roos, R. H. M. Taibah, S. Trincaz-Duvoid & J. Zahreddine
Department of Physics, University of Pennsylvania, Philadelphia, PA, USA
S. Chen, R. A. Creager, J. R. Dandoy, G. I. Dyckes, L. Flores, J. G. Heinlein, J. Kroll, E. Lipeles, J. Machado Miguens, B. J. Rosser, L. Schaefer, J. D. Shahinian, E. Thomson, H. H. Williams & R. Xu
Konstantinov Nuclear Physics Institute of National Research Centre “Kurchatov Institute”, PNPI, St. Petersburg, Russia
S. Barsov, A. Ezhilov, O. L. Fedin, V. Gratchev, M. Levchenko, V. P. Maleev, I. Naryshkin, D. Pudzha, V. A. Schegelsky & V. Solovyev
Department of Physics and Astronomy, University of Pittsburgh, Pittsburgh, PA, USA
M. Bandieramonte, R. Bi, R. M. Bianchi, J. Boudreau, H. Cai, B. T. Carlson, T. M. Hong, S. A. M. Merkt, J. Mueller, A. A. Myers, C. W. Ng, H. J. Stelzer & N. B. Suarez
Laboratório de Instrumentação e Física Experimental de Partículas - LIP, Lisbon, Portugal
J. A. Aguilar-Saavedra, S. P. Amor Dos Santos, N. F. Castro, P. Conde Muiño, T. Dias Do Vale, G. Evans, M. C. N. Fiolhais, A. Gomes, R. Gonçalo, A. Maio, E. D. Mendes Gouveia, A. L. Moreira De Carvalho, I. Ochoa, L. F. Oleiro Seabra, A. Onofre, R. Pedro, A. P. Pereira Peixoto, H. Santos, J. G. Saraiva, M. Stolarski, A. Tavares Delgado, F. Veloso & H. Wolters
Departamento de Física, Faculdade de Ciências, Universidade de Lisboa, Lisbon, Portugal
A. Gomes, A. Maio & H. Santos
Departamento de Física, Universidade de Coimbra, Coimbra, Portugal
M. C. N. Fiolhais, R. Gonçalo, F. Veloso & H. Wolters
Centro de Física Nuclear da Universidade de Lisboa, Lisbon, Portugal
A. Maio & J. G. Saraiva
Departamento de Física, Universidade do Minho, Braga, Portugal
N. F. Castro, E. D. Mendes Gouveia & A. Onofre
Departamento de Física Teórica y del Cosmos, Universidad de Granada, Granada, Spain
J. A. Aguilar-Saavedra
Instituto Superior Técnico, Universidade de Lisboa, Lisbon, Portugal
P. Conde Muiño
Institute of Physics of the Czech Academy of Sciences, Prague, Czech Republic
J. Chudoba, J. Hejbal, O. Hladik, P. Jacka, O. Kepka, J. Kroll, A. Kupco, V. Latonova, M. Lokajicek, R. Lysak, M. Marcisovsky, M. Mikestikova, S. Nemecek, O. Penc, P. Sicho, P. Staroba, M. Svatos & M. Tasevsky
Czech Technical University in Prague, Prague, Czech Republic
B. Ali, K. Augsten, B. Bergmann, T. R. V. Billoud, M. Havranek, Z. Hubacek, M. Myska, V. Petousis, S. Pospisil, K. Smolek, A. Sopczak, V. Vacek, P. Vokac & V. Vrba
Charles University, Faculty of Mathematics and Physics, Prague, Czech Republic
I. Carli, T. Davidek, J. Dolejsi, Z. Dolezal, J. Faltova, P. Kodys, M. Krivos, R. Leitner, K. Petukhova, V. Pleskot, R. Polifka, P. Reznicek, M. Rybar, D. Scheirich, M. Spousta, M. Sykora, T. Sykora, P. Tas, S. Todorova-Nova & V. Vorobel
Particle Physics Department, Rutherford Appleton Laboratory, Didcot, UK
T. Adye, J. T. Baines, B. M. Barnett, W. Buttinger, J. Dopke, D. Emeliyanov, B. J. Gallop, C. N. P. Gee, S. J. Haywood, J. Kirk, S. Martin-Haugh, S. J. McMahon, R. P. Middleton, W. J. Murray, R. E. Owen, P. W. Phillips, D. P. C. Sankey, C. Sawyer, B. H. Smart, W. Song, J. Walder & M. Wielers
IRFU, CEA, Université Paris-Saclay, Gif-sur-Yvette, France
N. Andari, H. Bachacou, F. Balli, F. Bauer, N. Besson, M. Boonekamp, T. J. A. Chevalérias, L. Chevalier, F. Deliot, A. Formica, P. F. Giraud, C. Guyot, S. Hassani, F. Jeanneau, T. Kawamoto, M. Khandoga, M. Kolb, J. F. Laporte, B. Mansoulie, J-P. Meyer, T. Moskalets, R. Nicolaidou, A. Ouraou, A. Peyaud, L. Schoeffel, Ph. Schune, Ph. Schwemling, M. Vandenbroucke & T. Xu
Santa Cruz Institute for Particle Physics, University of California Santa Cruz, Santa Cruz, CA, USA
A. A. Affolder, M. Battaglia, C. M. Gee, M. Gignac, A. A. Grillo, M. Hance, C. M. Helling, N. J. Kang, S. M. Mazza, J. Nielsen, J. M. P. Pasner, H.F-W. Sadrozinski, B. A. Schumm, A. Sciandra, A. Seiden, G. H. Stark, N. L. Woods & Y. Zhao
Departamento de Física, Pontificia Universidad Católica de Chile, Santiago, Chile
M. A. Diaz, F. M. Garay Walls, J. P. Ochoa-Ricoux & S. A. Olivares Pino
Universidad Andres Bello, Department of Physics, Santiago, Chile
S. Kuleshov
Instituto de Alta Investigación, Universidad de Tarapacá, Santiago, Chile
T. Lagouri
Departamento de Física, Universidad Técnica Federico Santa María, Valparaíso, Chile
W. K. Brooks, E. Carquin, R. Pezoa, C. M. Robles Gajardo, R. A. Rojas & N. Viaux Maira
Universidade Federal de São João del Rei (UFSJ), São João del Rei, Brazil
M. A. B. Do Vale
Department of Physics, University of Washington, Seattle, WA, USA
C. Alpigiani, S. J. Gasiorowski, A. G. Goussiou, S.-C. Hsu, A. K. Kvam, K. Li, H. J. Lubatti, M. L. Proffitt, J. Schaarschmidt, W. Su & G. Watts
Department of Physics and Astronomy, University of Sheffield, Sheffield, UK
C. Anastopoulos, M. T. Anthony, D. Costanzo, I. Dawson, A. Fell, J. J. Hall, M. C. Hodgkinson, P. Johansson, E. V. Korolkova, V. V. Kostyukhin, K. Lohwasser, A. Lopez Solis, C. M. Macdonald, J. C. MacDonald, H. Mildner, T. D. Powell, N. P. Readioff, E. M. Rüttinger, K. A. Saoucha, P. Sommer, D. R. Tovey, T. Vickey, O. E. Vickey Boeriu & T. G. Zorbas
Department of Physics, Shinshu University, Nagano, Japan
Y. Hasegawa, K. Kawade & T. Takeshita
Department Physik, Universität Siegen, Siegen, Germany
B. Batool, P. Buchholz, C. Diez Pardos, I. Fleck, M. Ghneimat, I. Ibragimov, J. K. R. Meshreki, A. Rej, W. Walkowiak & M. Ziolkowski
Department of Physics, Simon Fraser University, Burnaby, BC, Canada
H. Bahrasemani, M. Danninger, E. Drechsler, E. Dreyer, B. P. Jaeger, K. Lehmann, B. X. Liu, J. Llorente Merino, A. Montalbano, D. Mori, D. C. O’Neil, A. Poley, S. Singh, B. Stelzer & M. C. Vetterli
SLAC National Accelerator Laboratory, Stanford, CA, USA
T. Barklow, R. Bartoldus, C. Bernius, L. Bryngemark, V. M. M. Cairo, R. M. D. Carney, A. R. Cukierman, N. Garelli, P. Grenier, N. M. Hartman, R. Hyneman, Z. Jiang, M. Kagan, M. Kocian, J. Pearkes, M. Safdari, A. Salnikov, A. Schwartzman, S. Sevova, D. Su, R. Teixeira De Lima, L. Tompkins, C. Vernieri, M. Wittgen, Z. Xu & C. Young
Physics Department, Royal Institute of Technology, Stockholm, Sweden
B. Lund-Jensen, C. C. Ohm, G. Ripellino, D. R. Shope & J. Strandberg
Departments of Physics and Astronomy, Stony Brook University, Stony Brook, NY, USA
C. P. Bee, A. Behera, S. Bhatta, Q. Buat, J. Hobbs, J. Jia, I. Luise, G. Piacquadio, M. Rijssenbeek, R. D. Schamberger, V. Tsiskaridze, D. Tsybychev & M. Zhou
Department of Physics and Astronomy, University of Sussex, Brighton, UK
N. L. Abraham, B. M. M. Allbrooke, M. A. Aparo, L. Asquith, A. Cerri, A. De Santo, M. O. Evans, M. Grandi, S. D. Jones, D. Kelsey, D. M. Koeck, B. Safarzadeh Samani, F. Salvatore, K. Shaw, M. Spina, T. J. Stevenson, M. R. Sutton, F. Tresoldi, F. Trovato, I. Vivarelli, E. Winkels & I. **otidis
School of Physics, University of Sydney, Sydney, Australia
S. Balaji, C. J. E. Suster, K. E. Varvell & B. Yabsley
Institute of Physics, Academia Sinica, Taipei, Taiwan
S. Ali, A. Buzatu, S. Hou, S. C. Lee, R. Mazini, T. Varol & S. M. Wang
E. Andronikashvili Institute of Physics, Iv. Javakhishvili Tbilisi State University, Tbilisi, Georgia
J. Jejelava & E. G. Tskhadadze
High Energy Physics Institute, Tbilisi State University, Tbilisi, Georgia
B. Chargeishvili, T. Djobava, A. Durglishvili, J. Khubua, G. Mchedlidze, I. A. Minashvili, M. Mosidze & T. Zakareishvili
Department of Physics, Technion, Israel Institute of Technology, Haifa, Israel
H. Abreu, Y. Afik, R. Barnea, R. Brener, S. Istin, E. Kajomovitz, D. Melini, M. Morgenstern, Y. Rozen & S. Tarem
Raymond and Beverly Sackler School of Physics and Astronomy, Tel Aviv University, Tel Aviv, Israel
H. Abramowicz, M. J. Alconada Verzini, L. Barak, U. Barron, G. Bella, Y. Benhammou, T. Cao, H. Cohen, E. Etzion, G. Koren, M. S. Lutz, M. Pitt, D. Reikher, A. Soffer, J. Taenzer & N. M. Tamir
Department of Physics, Aristotle University of Thessaloniki, Thessaloniki, Greece
K. Bachas, S. Gkaitatzis, I. Karkanias, E. Kasimi, K. Kordas, C. Lampoudis, A. Leisos, I. M. Maniatis, I. Manthos, A. Marantis, I. Maznas, C. Petridou, D. Sampsonidis, A. Tsirigotis, M. Tsopoulou & S. Tzamarias
International Center for Elementary Particle Physics and Department of Physics, University of Tokyo, Tokyo, Japan
S. Asai, I. Chiu, Y. Enari, R. Iguchi, M. Ishino, M. Kaneda, T. Kishimoto, T. Kodama, T. Mashimo, T. Masubuchi, N. Matsuzawa, Y. Minegishi, T. Nakamura, T. Nobe, R. Oishi, Y. Okumura, M. Saito, T. Saito, H. Sakamoto, R. Sawada, J. Tanaka, G. Tateno, K. Terashi, K. Uchida, K. Uno, M. Yamatani, T. Yamazaki & Y. Yang
Graduate School of Science and Technology, Tokyo Metropolitan University, Tokyo, Japan
C. Fukunaga
Department of Physics, Tokyo Institute of Technology, Tokyo, Japan
T. Asawatavonvanich, Y. He, O. **nouchi, E. Kim, A. Kubota, M. Kuze, H. Oide, S. Shirabe & Y. Yamaguchi
Tomsk State University, Tomsk, Russia
M. Aliev, M. Didenko, V. V. Kostyukhin & O. Kuchinskaia
Department of Physics, University of Toronto, Toronto, ON, Canada
S. H. Abidi, L. Adamek, O. Arnaez, M. J. Basso, J. W. S. Carter, A. F. Casha, B. M. Ciungu, K. J. R. Cormier, K. Dette, R. Di Sipio, J. H. Foo, C. A. Garner, N. Giangiacomi, Y. F. Han, N. Ilic, S. Ketabchi Haghighat, D. P. Kisliuk, P. Krieger, H. Y. Meng, A. Milic, O. Miu, M. L. Ojeda, R. S. Orr, T. H. Park, P. Savard, P. Sinervo, R. J. Teuscher, W. Trischuk & L. M. Veloce
TRIUMF, Vancouver, BC, Canada
E. M. Carlson, S. V. Chekulaev, N. P. Hessey, K. Iordanidou, L. L. Kurchaninov, B. Lefebvre, E. Perez Codina, A. Poley, B. Stelzer, O. Stelzer-Chilton, M. Swiatlowski, R. Tafirout, I. M. Trigger & M. Valente
Department of Physics and Astronomy, York University, Toronto, ON, Canada
C. David, M. J. Kareem, A. M. Rodríguez Vera, W. Y. Song & W. Taylor
Division of Physics and Tomonaga Center for the History of the Universe, Faculty of Pure and Applied Sciences, University of Tsukuba, Tsukuba, Japan
K. Hara, S. Hirose, H. Okawa, K. Sato, F. Ukegawa, S. Wada & R. Wakasa
Department of Physics and Astronomy, Tufts University, Medford, MA, USA
P. H. Beauchemin, V. Croft, A. S. Drobac, F. I. Kaya, K. Sliwa & H. Son
Department of Physics and Astronomy, University of California Irvine, Irvine, CA, USA
A. Armstrong, T. Cuhadar Donszelmann, M. J. Fenton, J. Gramling, A. J. Lankford, Y. W. Y. Ng, K. Ntekas, M. J. R. Olsson, M. Schernau, A. Taffard, G. Unel & D. Whiteson
Department of Physics and Astronomy, University of Uppsala, Uppsala, Sweden
E. M. Asimakopoulou, E. Bergeaas Kuutmann, P. Bokan, R. Brenner, T. Ekelof, V. Ellajosyula, M. Ellert, A. Ferrari, R. Gonzalez Suarez, M. F. Isacson & M. U. F. Martensson
Department of Physics, University of Illinois, Urbana, IL, USA
M. Atkinson, V. R. Bailey, Y. Cao, M. Feickert, B. H. Hooberman, Y. P. Kulinich, T. M. Liss, J. D. Long, M. S. Neubauer, M. W. Phipps, A. Puri, T. T. Rinn, A. M. Sickles, S. Tapia Araya, J. C. Zeng, M. Zhang & D. Zhong
Instituto de Física Corpuscular (IFIC), Centro Mixto Universidad de Valencia, CSIC, Valencia, Spain
J. A. Aparisi Pozo, A. J. Bailey, S. Cabrera Urbán, F. Cardillo, F. L. Castillo, V. Castillo Gimenez, M. J. Costa, C. Escobar, O. Estrada Pastor, A. Ferrer, L. Fiorini, E. Fullana Torregrosa, J. Fuster, C. García, J. E. García Navarro, S. González de la Hoz, G. R. Gonzalvo Rodriguez, J. G. R. Guerrero Rojas, E. Higón-Rodriguez, C. Lacasta, J. J. Lozano Bahilo, J. Mamuzic, S. Marti-Garcia, P. Martinez Agullo, V. A. Mitsou, M. Moreno Llácer, J. Poveda, A. Prades Ibanez, S. Rodriguez Bosca, A. Ruiz-Martinez, P. Sabatini, J. Salt, J. Sánchez, A. Santra, I. Sayago Galvan, U. Soldevila, E. Torró Pastor, A. Valero, J. A. Valls Ferrer, M. Villaplana Perez & M. Vos
Department of Physics, University of British Columbia, Vancouver, BC, Canada
F. Cormier, W. Fedorko, C. Gay, C. Gubbels, R. L. Hayes, E. E. Khoda, A. Lister, R. Newhouse, D. A. Trischuk & V. W. S. Wong
Department of Physics and Astronomy, University of Victoria, Victoria, BC, Canada
J. Albert, C. R. Anelli, E. M. Carlson, Y. H. Chiu, M. Ghasemi Bostanabad, K. Hamano, E. F. Kay, R. Keeler, R. Kowalewski, M. Lefebvre, D. M. Mac Donell, K. D. McLean, R. A. McPherson, J. C. Rivera Vergara, M. J. Shroff, R. Sobie & G. A. Vasquez
Fakultät für Physik und Astronomie, Julius-Maximilians-Universität Würzburg, Würzburg, Germany
D. S. Bhattacharya, M. Haleem, G. Khoriauli, R. Ströhmer, T. Swirski & T. Trefzger
Department of Physics, University of Warwick, Coventry, UK
K. Becker, G. Facini, P. F. Harrison, E. Jones, T. A. Martin, C. J. McNicol, W. J. Murray, M. Spangenberg & L. **a
Waseda University, Tokyo, Japan
T. Kaji, T. Mitani, M. Morinaga, T. Nitta, Y. Shimogama & K. Yorita
Department of Particle Physics and Astrophysics, Weizmann Institute of Science, Rehovot, Israel
P. Balek, M. Birman, S. Bressler, Z. H. Citron, E. Duchovni, M. Dumancic, S. Ganguly, E. Gross, N. Hod, A. Ivina, T. Jakoubek, L. J. Levinson, G. Mikenberg, A. Milov, B. Parida, I. Ravinovich, D. Shaked Renous, M. Shehade, J. Shlomi, E. Shulga, V. Smakhtin & D. Turgeman
Department of Physics, University of Wisconsin, Madison, WI, USA
Sw. Banerjee, D. Biswas, J. Chan, W. Guan, L. S. Kaplan, A. Pathak, M. Jr. Silva, A. Z. Wang, W. Wiedenmann, S. L. Wu, R. Zhang, C. Zhou & G. Zobernig
Fakultät für Mathematik und Naturwissenschaften, Fachgruppe Physik, Bergische Universität Wuppertal, Wuppertal, Germany
V. A. Austrup, T. A. Beermann, O. Bessidskaia Bylund, G. Brandt, C. Dülsen, F. Ellinghaus, M. Errenst, T. Flick, G. Gilles, T. Harenberg, D. Hirschbuehl, G. Jäkel, S. Kersten, M. Neumann, J. Roggel, A. Sahu, M. Sandhoff, F. Schroeder, M. Vogel, W. Wagner, S. Wahdan & C. Zeitnitz
Department of Physics, Yale University, New Haven, CT, USA
O. K. Baker, E. G. Castiglia, S. Demers, M. Pettee, C. O. Shimmin & P. Tipton
Borough of Manhattan Community College, City University of New York, New York, NY, USA
M. C. N. Fiolhais
Center for High Energy Physics, Peking University, China
S. Li
Centro Studi e Ricerche Enrico Fermi, Rome, Italy
I. Gnesi
CERN, Geneva, Switzerland
P. Jenni
CPPM, Aix-Marseille Université, CNRS/IN2P3, Marseille, France
G. Tarna
Département de Physique Nucléaire et Corpusculaire, Université de Genève, Geneva, Switzerland
M. Nessi
Departament de Fisica de la Universitat Autonoma de Barcelona, Barcelona, Spain
M. P. Casado
Department of Financial and Management Engineering, University of the Aegean, Chios, Greece
I. Gkialas & K. Papageorgiou
Department of Physics and Astronomy, Michigan State University, East Lansing, MI, USA
R. Yuan
Department of Physics and Astronomy, University of Louisville, Louisville, KY, USA
Sw. Banerjee, D. Biswas & A. Pathak
Department of Physics, Ben Gurion University of the Negev, Beer Sheva, Israel
Z. H. Citron
Department of Physics, California State University, East Bay, USA
K. Grimm
Department of Physics, California State University, Fresno, USA
Y. S. Gao
Department of Physics, California State University, Sacramento, USA
J. Moss
Department of Physics, King’s College London, London, UK
B. S. Acharya
Department of Physics, St. Petersburg State Polytechnical University, St. Petersburg, Russia
O. L. Fedin
Department of Physics, University of Fribourg, Fribourg, Switzerland
H. P. Beck
Dipartimento di Matematica, Informatica e Fisica, Università di Udine, Udine, Italy
J. Magro
Faculty of Physics, M.V. Lomonosov Moscow State University, Moscow, Russia
L. N. Smirnova
Giresun University, Faculty of Engineering, Giresun, Turkey
I. Turk Cakir
Graduate School of Science, Osaka University, Osaka, Japan
K. Hanagaki
Hellenic Open University, Patras, Greece
A. Leisos & A. Tsirigotis
Institucio Catalana de Recerca i Estudis Avancats, ICREA, Barcelona, Spain
S. Grinstein, A. Juste Rozas & M. Martinez
Institut für Experimentalphysik, Universität Hamburg, Hamburg, Germany
K. Tackmann
Institute for Nuclear Research and Nuclear Energy (INRNE) of the Bulgarian Academy of Sciences, Sofia, Bulgaria
M. Shiyakova
Institute for Particle and Nuclear Physics, Wigner Research Centre for Physics, Budapest, Hungary
J. Toth
Institute of Particle Physics (IPP), Montreal, Canada
F. Corriveau, N. Ilic, R. A. McPherson, S. H. Robertson, R. Sobie & R. J. Teuscher
Institute of Physics, Azerbaijan Academy of Sciences, Baku, Azerbaijan
N. Huseynov & N. Javadov
Instituto de Fisica Teorica, IFT-UAM/CSIC, Madrid, Spain
J. A. Aguilar-Saavedra
Istanbul University, Department of Physics, Istanbul, Turkey
A. Adiguzel
Joint Institute for Nuclear Research, Dubna, Russia
Y. Kulchitsky & P. V. Tsiareshka
Moscow Institute of Physics and Technology State University, Dolgoprudny, Russia
G. A. Chelkov, A. G. Myagkov, V. Nikolaenko & A. M. Zaitsev
National Research Nuclear University MEPhI, Moscow, Russia
V. O. Tikhomirov
Physics Department, An-Najah National University, Nablus, Palestine
A. Bassalat
Physikalisches Institut, Albert-Ludwigs-Universität Freiburg, Freiburg, Germany
B. Heinemann
The City College of New York, New York, NY, USA
T. M. Liss
TRIUMF, Vancouver, BC, Canada
G. Azuelos, D. M. Gingrich, F. G. Oakham, P. Savard & M. C. Vetterli
Universita di Napoli Parthenope, Naples, Italy
F. Conventi
University of Chinese Academy of Sciences (UCAS), Bei**g, China
Y. F. Hu
- G. Aad
You can also search for this author in PubMed Google Scholar
- B. Abbott
You can also search for this author in PubMed Google Scholar
- D. C. Abbott
You can also search for this author in PubMed Google Scholar
- A. Abed Abud
You can also search for this author in PubMed Google Scholar
- K. Abeling
You can also search for this author in PubMed Google Scholar
- D. K. Abhayasinghe
You can also search for this author in PubMed Google Scholar
- S. H. Abidi
You can also search for this author in PubMed Google Scholar
- O. S. AbouZeid
You can also search for this author in PubMed Google Scholar
- N. L. Abraham
You can also search for this author in PubMed Google Scholar
- H. Abramowicz
You can also search for this author in PubMed Google Scholar
- H. Abreu
You can also search for this author in PubMed Google Scholar
- Y. Abulaiti
You can also search for this author in PubMed Google Scholar
- B. S. Acharya
You can also search for this author in PubMed Google Scholar
- B. Achkar
You can also search for this author in PubMed Google Scholar
- L. Adam
You can also search for this author in PubMed Google Scholar
- C. Adam Bourdarios
You can also search for this author in PubMed Google Scholar
- L. Adamczyk
You can also search for this author in PubMed Google Scholar
- L. Adamek
You can also search for this author in PubMed Google Scholar
- J. Adelman
You can also search for this author in PubMed Google Scholar
- A. Adiguzel
You can also search for this author in PubMed Google Scholar
- S. Adorni
You can also search for this author in PubMed Google Scholar
- T. Adye
You can also search for this author in PubMed Google Scholar
- A. A. Affolder
You can also search for this author in PubMed Google Scholar
- Y. Afik
You can also search for this author in PubMed Google Scholar
- C. Agapopoulou
You can also search for this author in PubMed Google Scholar
- M. N. Agaras
You can also search for this author in PubMed Google Scholar
- A. Aggarwal
You can also search for this author in PubMed Google Scholar
- C. Agheorghiesei
You can also search for this author in PubMed Google Scholar
- J. A. Aguilar-Saavedra
You can also search for this author in PubMed Google Scholar
- A. Ahmad
You can also search for this author in PubMed Google Scholar
- F. Ahmadov
You can also search for this author in PubMed Google Scholar
- W. S. Ahmed
You can also search for this author in PubMed Google Scholar
- X. Ai
You can also search for this author in PubMed Google Scholar
- G. Aielli
You can also search for this author in PubMed Google Scholar
- S. Akatsuka
You can also search for this author in PubMed Google Scholar
- M. Akbiyik
You can also search for this author in PubMed Google Scholar
- T. P. A. Åkesson
You can also search for this author in PubMed Google Scholar
- E. Akilli
You can also search for this author in PubMed Google Scholar
- A. V. Akimov
You can also search for this author in PubMed Google Scholar
- K. Al Khoury
You can also search for this author in PubMed Google Scholar
- G. L. Alberghi
You can also search for this author in PubMed Google Scholar
- J. Albert
You can also search for this author in PubMed Google Scholar
- M. J. Alconada Verzini
You can also search for this author in PubMed Google Scholar
- S. Alderweireldt
You can also search for this author in PubMed Google Scholar
- M. Aleksa
You can also search for this author in PubMed Google Scholar
- I. N. Aleksandrov
You can also search for this author in PubMed Google Scholar
- C. Alexa
You can also search for this author in PubMed Google Scholar
- T. Alexopoulos
You can also search for this author in PubMed Google Scholar
- A. Alfonsi
You can also search for this author in PubMed Google Scholar
- F. Alfonsi
You can also search for this author in PubMed Google Scholar
- M. Alhroob
You can also search for this author in PubMed Google Scholar
- B. Ali
You can also search for this author in PubMed Google Scholar
- S. Ali
You can also search for this author in PubMed Google Scholar
- M. Aliev
You can also search for this author in PubMed Google Scholar
- G. Alimonti
You can also search for this author in PubMed Google Scholar
- C. Allaire
You can also search for this author in PubMed Google Scholar
- B. M. M. Allbrooke
You can also search for this author in PubMed Google Scholar
- B. W. Allen
You can also search for this author in PubMed Google Scholar
- P. P. Allport
You can also search for this author in PubMed Google Scholar
- A. Aloisio
You can also search for this author in PubMed Google Scholar
- F. Alonso
You can also search for this author in PubMed Google Scholar
- C. Alpigiani
You can also search for this author in PubMed Google Scholar
- E. Alunno Camelia
You can also search for this author in PubMed Google Scholar
- M. Alvarez Estevez
You can also search for this author in PubMed Google Scholar
- M. G. Alviggi
You can also search for this author in PubMed Google Scholar
- Y. Amaral Coutinho
You can also search for this author in PubMed Google Scholar
- A. Ambler
You can also search for this author in PubMed Google Scholar
- L. Ambroz
You can also search for this author in PubMed Google Scholar
- C. Amelung
You can also search for this author in PubMed Google Scholar
- D. Amidei
You can also search for this author in PubMed Google Scholar
- S. P. Amor Dos Santos
You can also search for this author in PubMed Google Scholar
- S. Amoroso
You can also search for this author in PubMed Google Scholar
- C. S. Amrouche
You can also search for this author in PubMed Google Scholar
- F. An
You can also search for this author in PubMed Google Scholar
- C. Anastopoulos
You can also search for this author in PubMed Google Scholar
- N. Andari
You can also search for this author in PubMed Google Scholar
- T. Andeen
You can also search for this author in PubMed Google Scholar
- J. K. Anders
You can also search for this author in PubMed Google Scholar
- S. Y. Andrean
You can also search for this author in PubMed Google Scholar
- A. Andreazza
You can also search for this author in PubMed Google Scholar
- V. Andrei
You can also search for this author in PubMed Google Scholar
- C. R. Anelli
You can also search for this author in PubMed Google Scholar
- S. Angelidakis
You can also search for this author in PubMed Google Scholar
- A. Angerami
You can also search for this author in PubMed Google Scholar
- A. V. Anisenkov
You can also search for this author in PubMed Google Scholar
- A. Annovi
You can also search for this author in PubMed Google Scholar
- C. Antel
You can also search for this author in PubMed Google Scholar
- M. T. Anthony
You can also search for this author in PubMed Google Scholar
- E. Antipov
You can also search for this author in PubMed Google Scholar
- M. Antonelli
You can also search for this author in PubMed Google Scholar
- D. J. A. Antrim
You can also search for this author in PubMed Google Scholar
- F. Anulli
You can also search for this author in PubMed Google Scholar
- M. Aoki
You can also search for this author in PubMed Google Scholar
- J. A. Aparisi Pozo
You can also search for this author in PubMed Google Scholar
- M. A. Aparo
You can also search for this author in PubMed Google Scholar
- L. Aperio Bella
You can also search for this author in PubMed Google Scholar
- N. Aranzabal
You can also search for this author in PubMed Google Scholar
- V. Araujo Ferraz
You can also search for this author in PubMed Google Scholar
- R. Araujo Pereira
You can also search for this author in PubMed Google Scholar
- C. Arcangeletti
You can also search for this author in PubMed Google Scholar
- A. T. H. Arce
You can also search for this author in PubMed Google Scholar
- J-F. Arguin
You can also search for this author in PubMed Google Scholar
- S. Argyropoulos
You can also search for this author in PubMed Google Scholar
- J.-H. Arling
You can also search for this author in PubMed Google Scholar
- A. J. Armbruster
You can also search for this author in PubMed Google Scholar
- A. Armstrong
You can also search for this author in PubMed Google Scholar
- O. Arnaez
You can also search for this author in PubMed Google Scholar
- H. Arnold
You can also search for this author in PubMed Google Scholar
- Z. P. Arrubarrena Tame
You can also search for this author in PubMed Google Scholar
- G. Artoni
You can also search for this author in PubMed Google Scholar
- H. Asada
You can also search for this author in PubMed Google Scholar
- K. Asai
You can also search for this author in PubMed Google Scholar
- S. Asai
You can also search for this author in PubMed Google Scholar
- T. Asawatavonvanich
You can also search for this author in PubMed Google Scholar
- N. Asbah
You can also search for this author in PubMed Google Scholar
- E. M. Asimakopoulou
You can also search for this author in PubMed Google Scholar
- L. Asquith
You can also search for this author in PubMed Google Scholar
- J. Assahsah
You can also search for this author in PubMed Google Scholar
- K. Assamagan
You can also search for this author in PubMed Google Scholar
- R. Astalos
You can also search for this author in PubMed Google Scholar
- R. J. Atkin
You can also search for this author in PubMed Google Scholar
- M. Atkinson
You can also search for this author in PubMed Google Scholar
- N. B. Atlay
You can also search for this author in PubMed Google Scholar
- H. Atmani
You can also search for this author in PubMed Google Scholar
- P. A. Atmasiddha
You can also search for this author in PubMed Google Scholar
- K. Augsten
You can also search for this author in PubMed Google Scholar
- V. A. Austrup
You can also search for this author in PubMed Google Scholar
- G. Avolio
You can also search for this author in PubMed Google Scholar
- M. K. Ayoub
You can also search for this author in PubMed Google Scholar
- G. Azuelos
You can also search for this author in PubMed Google Scholar
- D. Babal
You can also search for this author in PubMed Google Scholar
- H. Bachacou
You can also search for this author in PubMed Google Scholar
- K. Bachas
You can also search for this author in PubMed Google Scholar
- F. Backman
You can also search for this author in PubMed Google Scholar
- P. Bagnaia
You can also search for this author in PubMed Google Scholar
- M. Bahmani
You can also search for this author in PubMed Google Scholar
- H. Bahrasemani
You can also search for this author in PubMed Google Scholar
- A. J. Bailey
You can also search for this author in PubMed Google Scholar
- V. R. Bailey
You can also search for this author in PubMed Google Scholar
- J. T. Baines
You can also search for this author in PubMed Google Scholar
- C. Bakalis
You can also search for this author in PubMed Google Scholar
- O. K. Baker
You can also search for this author in PubMed Google Scholar
- P. J. Bakker
You can also search for this author in PubMed Google Scholar
- E. Bakos
You can also search for this author in PubMed Google Scholar
- D. Bakshi Gupta
You can also search for this author in PubMed Google Scholar
- S. Balaji
You can also search for this author in PubMed Google Scholar
- R. Balasubramanian
You can also search for this author in PubMed Google Scholar
- E. M. Baldin
You can also search for this author in PubMed Google Scholar
- P. Balek
You can also search for this author in PubMed Google Scholar
- F. Balli
You can also search for this author in PubMed Google Scholar
- W. K. Balunas
You can also search for this author in PubMed Google Scholar
- J. Balz
You can also search for this author in PubMed Google Scholar
- E. Banas
You can also search for this author in PubMed Google Scholar
- M. Bandieramonte
You can also search for this author in PubMed Google Scholar
- A. Bandyopadhyay
You can also search for this author in PubMed Google Scholar
- Sw. Banerjee
You can also search for this author in PubMed Google Scholar
- L. Barak
You can also search for this author in PubMed Google Scholar
- W. M. Barbe
You can also search for this author in PubMed Google Scholar
- E. L. Barberio
You can also search for this author in PubMed Google Scholar
- D. Barberis
You can also search for this author in PubMed Google Scholar
- M. Barbero
You can also search for this author in PubMed Google Scholar
- G. Barbour
You can also search for this author in PubMed Google Scholar
- T. Barillari
You can also search for this author in PubMed Google Scholar
- M-S. Barisits
You can also search for this author in PubMed Google Scholar
- J. Barkeloo
You can also search for this author in PubMed Google Scholar
- T. Barklow
You can also search for this author in PubMed Google Scholar
- R. Barnea
You can also search for this author in PubMed Google Scholar
- B. M. Barnett
You can also search for this author in PubMed Google Scholar
- R. M. Barnett
You can also search for this author in PubMed Google Scholar
- Z. Barnovska-Blenessy
You can also search for this author in PubMed Google Scholar
- A. Baroncelli
You can also search for this author in PubMed Google Scholar
- G. Barone
You can also search for this author in PubMed Google Scholar
- A. J. Barr
You can also search for this author in PubMed Google Scholar
- L. Barranco Navarro
You can also search for this author in PubMed Google Scholar
- F. Barreiro
You can also search for this author in PubMed Google Scholar
- J. Barreiro Guimarães da Costa
You can also search for this author in PubMed Google Scholar
- U. Barron
You can also search for this author in PubMed Google Scholar
- S. Barsov
You can also search for this author in PubMed Google Scholar
- F. Bartels
You can also search for this author in PubMed Google Scholar
- R. Bartoldus
You can also search for this author in PubMed Google Scholar
- G. Bartolini
You can also search for this author in PubMed Google Scholar
- A. E. Barton
You can also search for this author in PubMed Google Scholar
- P. Bartos
You can also search for this author in PubMed Google Scholar
- A. Basalaev
You can also search for this author in PubMed Google Scholar
- A. Basan
You can also search for this author in PubMed Google Scholar
- A. Bassalat
You can also search for this author in PubMed Google Scholar
- M. J. Basso
You can also search for this author in PubMed Google Scholar
- R. L. Bates
You can also search for this author in PubMed Google Scholar
- S. Batlamous
You can also search for this author in PubMed Google Scholar
- J. R. Batley
You can also search for this author in PubMed Google Scholar
- B. Batool
You can also search for this author in PubMed Google Scholar
- M. Battaglia
You can also search for this author in PubMed Google Scholar
- M. Bauce
You can also search for this author in PubMed Google Scholar
- F. Bauer
You can also search for this author in PubMed Google Scholar
- P. Bauer
You can also search for this author in PubMed Google Scholar
- H. S. Bawa
You can also search for this author in PubMed Google Scholar
- A. Bayirli
You can also search for this author in PubMed Google Scholar
- J. B. Beacham
You can also search for this author in PubMed Google Scholar
- T. Beau
You can also search for this author in PubMed Google Scholar
- P. H. Beauchemin
You can also search for this author in PubMed Google Scholar
- F. Becherer
You can also search for this author in PubMed Google Scholar
- P. Bechtle
You can also search for this author in PubMed Google Scholar
- H. C. Beck
You can also search for this author in PubMed Google Scholar
- H. P. Beck
You can also search for this author in PubMed Google Scholar
- K. Becker
You can also search for this author in PubMed Google Scholar
- C. Becot
You can also search for this author in PubMed Google Scholar
- A. Beddall
You can also search for this author in PubMed Google Scholar
- A. J. Beddall
You can also search for this author in PubMed Google Scholar
- V. A. Bednyakov
You can also search for this author in PubMed Google Scholar
- M. Bedognetti
You can also search for this author in PubMed Google Scholar
- C. P. Bee
You can also search for this author in PubMed Google Scholar
- T. A. Beermann
You can also search for this author in PubMed Google Scholar
- M. Begalli
You can also search for this author in PubMed Google Scholar
- M. Begel
You can also search for this author in PubMed Google Scholar
- A. Behera
You can also search for this author in PubMed Google Scholar
- J. K. Behr
You can also search for this author in PubMed Google Scholar
- F. Beisiegel
You can also search for this author in PubMed Google Scholar
- M. Belfkir
You can also search for this author in PubMed Google Scholar
- A. S. Bell
You can also search for this author in PubMed Google Scholar
- G. Bella
You can also search for this author in PubMed Google Scholar
- L. Bellagamba
You can also search for this author in PubMed Google Scholar
- A. Bellerive
You can also search for this author in PubMed Google Scholar
- P. Bellos
You can also search for this author in PubMed Google Scholar
- K. Beloborodov
You can also search for this author in PubMed Google Scholar
- K. Belotskiy
You can also search for this author in PubMed Google Scholar
- N. L. Belyaev
You can also search for this author in PubMed Google Scholar
- D. Benchekroun
You can also search for this author in PubMed Google Scholar
- N. Benekos
You can also search for this author in PubMed Google Scholar
- Y. Benhammou
You can also search for this author in PubMed Google Scholar
- D. P. Benjamin
You can also search for this author in PubMed Google Scholar
- M. Benoit
You can also search for this author in PubMed Google Scholar
- J. R. Bensinger
You can also search for this author in PubMed Google Scholar
- S. Bentvelsen
You can also search for this author in PubMed Google Scholar
- L. Beresford
You can also search for this author in PubMed Google Scholar
- M. Beretta
You can also search for this author in PubMed Google Scholar
- D. Berge
You can also search for this author in PubMed Google Scholar
- E. Bergeaas Kuutmann
You can also search for this author in PubMed Google Scholar
- N. Berger
You can also search for this author in PubMed Google Scholar
- B. Bergmann
You can also search for this author in PubMed Google Scholar
- L. J. Bergsten
You can also search for this author in PubMed Google Scholar
- J. Beringer
You can also search for this author in PubMed Google Scholar
- S. Berlendis
You can also search for this author in PubMed Google Scholar
- G. Bernardi
You can also search for this author in PubMed Google Scholar
- C. Bernius
You can also search for this author in PubMed Google Scholar
- F. U. Bernlochner
You can also search for this author in PubMed Google Scholar
- T. Berry
You can also search for this author in PubMed Google Scholar
- P. Berta
You can also search for this author in PubMed Google Scholar
- A. Berthold
You can also search for this author in PubMed Google Scholar
- I. A. Bertram
You can also search for this author in PubMed Google Scholar
- O. Bessidskaia Bylund
You can also search for this author in PubMed Google Scholar
- N. Besson
You can also search for this author in PubMed Google Scholar
- S. Bethke
You can also search for this author in PubMed Google Scholar
- A. Betti
You can also search for this author in PubMed Google Scholar
- A. J. Bevan
You can also search for this author in PubMed Google Scholar
- J. Beyer
You can also search for this author in PubMed Google Scholar
- S. Bhatta
You can also search for this author in PubMed Google Scholar
- D. S. Bhattacharya
You can also search for this author in PubMed Google Scholar
- P. Bhattarai
You can also search for this author in PubMed Google Scholar
- V. S. Bhopatkar
You can also search for this author in PubMed Google Scholar
- R. Bi
You can also search for this author in PubMed Google Scholar
- R. M. Bianchi
You can also search for this author in PubMed Google Scholar
- O. Biebel
You can also search for this author in PubMed Google Scholar
- D. Biedermann
You can also search for this author in PubMed Google Scholar
- R. Bielski
You can also search for this author in PubMed Google Scholar
- K. Bierwagen
You can also search for this author in PubMed Google Scholar
- N. V. Biesuz
You can also search for this author in PubMed Google Scholar
- M. Biglietti
You can also search for this author in PubMed Google Scholar
- T. R. V. Billoud
You can also search for this author in PubMed Google Scholar
- M. Bindi
You can also search for this author in PubMed Google Scholar
- A. Bingul
You can also search for this author in PubMed Google Scholar
- C. Bini
You can also search for this author in PubMed Google Scholar
- S. Biondi
You can also search for this author in PubMed Google Scholar
- C. J. Birch-sykes
You can also search for this author in PubMed Google Scholar
- M. Birman
You can also search for this author in PubMed Google Scholar
- T. Bisanz
You can also search for this author in PubMed Google Scholar
- J. P. Biswal
You can also search for this author in PubMed Google Scholar
- D. Biswas
You can also search for this author in PubMed Google Scholar
- A. Bitadze
You can also search for this author in PubMed Google Scholar
- C. Bittrich
You can also search for this author in PubMed Google Scholar
- K. Bjørke
You can also search for this author in PubMed Google Scholar
- T. Blazek
You can also search for this author in PubMed Google Scholar
- I. Bloch
You can also search for this author in PubMed Google Scholar
- C. Blocker
You can also search for this author in PubMed Google Scholar
- A. Blue
You can also search for this author in PubMed Google Scholar
- U. Blumenschein
You can also search for this author in PubMed Google Scholar
- G. J. Bobbink
You can also search for this author in PubMed Google Scholar
- V. S. Bobrovnikov
You can also search for this author in PubMed Google Scholar
- S. S. Bocchetta
You can also search for this author in PubMed Google Scholar
- D. Bogavac
You can also search for this author in PubMed Google Scholar
- A. G. Bogdanchikov
You can also search for this author in PubMed Google Scholar
- C. Bohm
You can also search for this author in PubMed Google Scholar
- V. Boisvert
You can also search for this author in PubMed Google Scholar
- P. Bokan
You can also search for this author in PubMed Google Scholar
- T. Bold
You can also search for this author in PubMed Google Scholar
- A. E. Bolz
You can also search for this author in PubMed Google Scholar
- M. Bomben
You can also search for this author in PubMed Google Scholar
- M. Bona
You can also search for this author in PubMed Google Scholar
- J. S. Bonilla
You can also search for this author in PubMed Google Scholar
- M. Boonekamp
You can also search for this author in PubMed Google Scholar
- C. D. Booth
You can also search for this author in PubMed Google Scholar
- A. G. Borbély
You can also search for this author in PubMed Google Scholar
- H. M. Borecka-Bielska
You can also search for this author in PubMed Google Scholar
- L. S. Borgna
You can also search for this author in PubMed Google Scholar
- A. Borisov
You can also search for this author in PubMed Google Scholar
- G. Borissov
You can also search for this author in PubMed Google Scholar
- D. Bortoletto
You can also search for this author in PubMed Google Scholar
- D. Boscherini
You can also search for this author in PubMed Google Scholar
- M. Bosman
You can also search for this author in PubMed Google Scholar
- J. D. Bossio Sola
You can also search for this author in PubMed Google Scholar
- K. Bouaouda
You can also search for this author in PubMed Google Scholar
- J. Boudreau
You can also search for this author in PubMed Google Scholar
- E. V. Bouhova-Thacker
You can also search for this author in PubMed Google Scholar
- D. Boumediene
You can also search for this author in PubMed Google Scholar
- A. Boveia
You can also search for this author in PubMed Google Scholar
- J. Boyd
You can also search for this author in PubMed Google Scholar
- D. Boye
You can also search for this author in PubMed Google Scholar
- I. R. Boyko
You can also search for this author in PubMed Google Scholar
- A. J. Bozson
You can also search for this author in PubMed Google Scholar
- J. Bracinik
You can also search for this author in PubMed Google Scholar
- N. Brahimi
You can also search for this author in PubMed Google Scholar
- G. Brandt
You can also search for this author in PubMed Google Scholar
- O. Brandt
You can also search for this author in PubMed Google Scholar
- F. Braren
You can also search for this author in PubMed Google Scholar
- B. Brau
You can also search for this author in PubMed Google Scholar
- J. E. Brau
You can also search for this author in PubMed Google Scholar
- W. D. Breaden Madden
You can also search for this author in PubMed Google Scholar
- K. Brendlinger
You can also search for this author in PubMed Google Scholar
- R. Brener
You can also search for this author in PubMed Google Scholar
- L. Brenner
You can also search for this author in PubMed Google Scholar
- R. Brenner
You can also search for this author in PubMed Google Scholar
- S. Bressler
You can also search for this author in PubMed Google Scholar
- B. Brickwedde
You can also search for this author in PubMed Google Scholar
- D. L. Briglin
You can also search for this author in PubMed Google Scholar
- D. Britton
You can also search for this author in PubMed Google Scholar
- D. Britzger
You can also search for this author in PubMed Google Scholar
- I. Brock
You can also search for this author in PubMed Google Scholar
- R. Brock
You can also search for this author in PubMed Google Scholar
- G. Brooijmans
You can also search for this author in PubMed Google Scholar
- W. K. Brooks
You can also search for this author in PubMed Google Scholar
- E. Brost
You can also search for this author in PubMed Google Scholar
- P. A. Bruckman de Renstrom
You can also search for this author in PubMed Google Scholar
- B. Brüers
You can also search for this author in PubMed Google Scholar
- D. Bruncko
You can also search for this author in PubMed Google Scholar
- A. Bruni
You can also search for this author in PubMed Google Scholar
- G. Bruni
You can also search for this author in PubMed Google Scholar
- M. Bruschi
You can also search for this author in PubMed Google Scholar
- N. Bruscino
You can also search for this author in PubMed Google Scholar
- L. Bryngemark
You can also search for this author in PubMed Google Scholar
- T. Buanes
You can also search for this author in PubMed Google Scholar
- Q. Buat
You can also search for this author in PubMed Google Scholar
- P. Buchholz
You can also search for this author in PubMed Google Scholar
- A. G. Buckley
You can also search for this author in PubMed Google Scholar
- I. A. Budagov
You can also search for this author in PubMed Google Scholar
- M. K. Bugge
You can also search for this author in PubMed Google Scholar
- O. Bulekov
You can also search for this author in PubMed Google Scholar
- B. A. Bullard
You can also search for this author in PubMed Google Scholar
- T. J. Burch
You can also search for this author in PubMed Google Scholar
- S. Burdin
You can also search for this author in PubMed Google Scholar
- C. D. Burgard
You can also search for this author in PubMed Google Scholar
- A. M. Burger
You can also search for this author in PubMed Google Scholar
- B. Burghgrave
You can also search for this author in PubMed Google Scholar
- J. T. P. Burr
You can also search for this author in PubMed Google Scholar
- C. D. Burton
You can also search for this author in PubMed Google Scholar
- J. C. Burzynski
You can also search for this author in PubMed Google Scholar
- V. Büscher
You can also search for this author in PubMed Google Scholar
- E. Buschmann
You can also search for this author in PubMed Google Scholar
- P. J. Bussey
You can also search for this author in PubMed Google Scholar
- J. M. Butler
You can also search for this author in PubMed Google Scholar
- C. M. Buttar
You can also search for this author in PubMed Google Scholar
- J. M. Butterworth
You can also search for this author in PubMed Google Scholar
- P. Butti
You can also search for this author in PubMed Google Scholar
- W. Buttinger
You can also search for this author in PubMed Google Scholar
- C. J. Buxo Vazquez
You can also search for this author in PubMed Google Scholar
- A. Buzatu
You can also search for this author in PubMed Google Scholar
- A. R. Buzykaev
You can also search for this author in PubMed Google Scholar
- G. Cabras
You can also search for this author in PubMed Google Scholar
- S. Cabrera Urbán
You can also search for this author in PubMed Google Scholar
- D. Caforio
You can also search for this author in PubMed Google Scholar
- H. Cai
You can also search for this author in PubMed Google Scholar
- V. M. M. Cairo
You can also search for this author in PubMed Google Scholar
- O. Cakir
You can also search for this author in PubMed Google Scholar
- N. Calace
You can also search for this author in PubMed Google Scholar
- P. Calafiura
You can also search for this author in PubMed Google Scholar
- G. Calderini
You can also search for this author in PubMed Google Scholar
- P. Calfayan
You can also search for this author in PubMed Google Scholar
- G. Callea
You can also search for this author in PubMed Google Scholar
- L. P. Caloba
You can also search for this author in PubMed Google Scholar
- A. Caltabiano
You can also search for this author in PubMed Google Scholar
- S. Calvente Lopez
You can also search for this author in PubMed Google Scholar
- D. Calvet
You can also search for this author in PubMed Google Scholar
- S. Calvet
You can also search for this author in PubMed Google Scholar
- T. P. Calvet
You can also search for this author in PubMed Google Scholar
- M. Calvetti
You can also search for this author in PubMed Google Scholar
- R. Camacho Toro
You can also search for this author in PubMed Google Scholar
- S. Camarda
You can also search for this author in PubMed Google Scholar
- D. Camarero Munoz
You can also search for this author in PubMed Google Scholar
- P. Camarri
You can also search for this author in PubMed Google Scholar
- M. T. Camerlingo
You can also search for this author in PubMed Google Scholar
- D. Cameron
You can also search for this author in PubMed Google Scholar
- C. Camincher
You can also search for this author in PubMed Google Scholar
- S. Campana
You can also search for this author in PubMed Google Scholar
- M. Campanelli
You can also search for this author in PubMed Google Scholar
- A. Camplani
You can also search for this author in PubMed Google Scholar
- V. Canale
You can also search for this author in PubMed Google Scholar
- A. Canesse
You can also search for this author in PubMed Google Scholar
- M. Cano Bret
You can also search for this author in PubMed Google Scholar
- J. Cantero
You can also search for this author in PubMed Google Scholar
- T. Cao
You can also search for this author in PubMed Google Scholar
- Y. Cao
You can also search for this author in PubMed Google Scholar
- M. Capua
You can also search for this author in PubMed Google Scholar
- R. Cardarelli
You can also search for this author in PubMed Google Scholar
- F. Cardillo
You can also search for this author in PubMed Google Scholar
- G. Carducci
You can also search for this author in PubMed Google Scholar
- I. Carli
You can also search for this author in PubMed Google Scholar
- T. Carli
You can also search for this author in PubMed Google Scholar
- G. Carlino
You can also search for this author in PubMed Google Scholar
- B. T. Carlson
You can also search for this author in PubMed Google Scholar
- E. M. Carlson
You can also search for this author in PubMed Google Scholar
- L. Carminati
You can also search for this author in PubMed Google Scholar
- R. M. D. Carney
You can also search for this author in PubMed Google Scholar
- S. Caron
You can also search for this author in PubMed Google Scholar
- E. Carquin
You can also search for this author in PubMed Google Scholar
- S. Carrá
You can also search for this author in PubMed Google Scholar
- G. Carratta
You can also search for this author in PubMed Google Scholar
- J. W. S. Carter
You can also search for this author in PubMed Google Scholar
- T. M. Carter
You can also search for this author in PubMed Google Scholar
- M. P. Casado
You can also search for this author in PubMed Google Scholar
- A. F. Casha
You can also search for this author in PubMed Google Scholar
- E. G. Castiglia
You can also search for this author in PubMed Google Scholar
- F. L. Castillo
You can also search for this author in PubMed Google Scholar
- L. Castillo Garcia
You can also search for this author in PubMed Google Scholar
- V. Castillo Gimenez
You can also search for this author in PubMed Google Scholar
- N. F. Castro
You can also search for this author in PubMed Google Scholar
- A. Catinaccio
You can also search for this author in PubMed Google Scholar
- J. R. Catmore
You can also search for this author in PubMed Google Scholar
- A. Cattai
You can also search for this author in PubMed Google Scholar
- V. Cavaliere
You can also search for this author in PubMed Google Scholar
- V. Cavasinni
You can also search for this author in PubMed Google Scholar
- E. Celebi
You can also search for this author in PubMed Google Scholar
- F. Celli
You can also search for this author in PubMed Google Scholar
- K. Cerny
You can also search for this author in PubMed Google Scholar
- A. S. Cerqueira
You can also search for this author in PubMed Google Scholar
- A. Cerri
You can also search for this author in PubMed Google Scholar
- L. Cerrito
You can also search for this author in PubMed Google Scholar
- F. Cerutti
You can also search for this author in PubMed Google Scholar
- A. Cervelli
You can also search for this author in PubMed Google Scholar
- S. A. Cetin
You can also search for this author in PubMed Google Scholar
- Z. Chadi
You can also search for this author in PubMed Google Scholar
- D. Chakraborty
You can also search for this author in PubMed Google Scholar
- J. Chan
You can also search for this author in PubMed Google Scholar
- W. S. Chan
You can also search for this author in PubMed Google Scholar
- W. Y. Chan
You can also search for this author in PubMed Google Scholar
- J. D. Chapman
You can also search for this author in PubMed Google Scholar
- B. Chargeishvili
You can also search for this author in PubMed Google Scholar
- D. G. Charlton
You can also search for this author in PubMed Google Scholar
- T. P. Charman
You can also search for this author in PubMed Google Scholar
- M. Chatterjee
You can also search for this author in PubMed Google Scholar
- C. C. Chau
You can also search for this author in PubMed Google Scholar
- S. Che
You can also search for this author in PubMed Google Scholar
- S. Chekanov
You can also search for this author in PubMed Google Scholar
- S. V. Chekulaev
You can also search for this author in PubMed Google Scholar
- G. A. Chelkov
You can also search for this author in PubMed Google Scholar
- B. Chen
You can also search for this author in PubMed Google Scholar
- C. Chen
You can also search for this author in PubMed Google Scholar
- C. H. Chen
You can also search for this author in PubMed Google Scholar
- H. Chen
You can also search for this author in PubMed Google Scholar
- H. Chen
You can also search for this author in PubMed Google Scholar
- J. Chen
You can also search for this author in PubMed Google Scholar
- J. Chen
You can also search for this author in PubMed Google Scholar
- J. Chen
You can also search for this author in PubMed Google Scholar
- S. Chen
You can also search for this author in PubMed Google Scholar
- S. J. Chen
You can also search for this author in PubMed Google Scholar
- X. Chen
You can also search for this author in PubMed Google Scholar
- Y. Chen
You can also search for this author in PubMed Google Scholar
- Y-H. Chen
You can also search for this author in PubMed Google Scholar
- H. C. Cheng
You can also search for this author in PubMed Google Scholar
- H. J. Cheng
You can also search for this author in PubMed Google Scholar
- A. Cheplakov
You can also search for this author in PubMed Google Scholar
- E. Cheremushkina
You can also search for this author in PubMed Google Scholar
- R. Cherkaoui El Moursli
You can also search for this author in PubMed Google Scholar
- E. Cheu
You can also search for this author in PubMed Google Scholar
- K. Cheung
You can also search for this author in PubMed Google Scholar
- T. J. A. Chevalérias
You can also search for this author in PubMed Google Scholar
- L. Chevalier
You can also search for this author in PubMed Google Scholar
- V. Chiarella
You can also search for this author in PubMed Google Scholar
- G. Chiarelli
You can also search for this author in PubMed Google Scholar
- G. Chiodini
You can also search for this author in PubMed Google Scholar
- A. S. Chisholm
You can also search for this author in PubMed Google Scholar
- A. Chitan
You can also search for this author in PubMed Google Scholar
- I. Chiu
You can also search for this author in PubMed Google Scholar
- Y. H. Chiu
You can also search for this author in PubMed Google Scholar
- M. V. Chizhov
You can also search for this author in PubMed Google Scholar
- K. Choi
You can also search for this author in PubMed Google Scholar
- A. R. Chomont
You can also search for this author in PubMed Google Scholar
- Y. Chou
You can also search for this author in PubMed Google Scholar
- Y. S. Chow
You can also search for this author in PubMed Google Scholar
- L. D. Christopher
You can also search for this author in PubMed Google Scholar
- M. C. Chu
You can also search for this author in PubMed Google Scholar
- X. Chu
You can also search for this author in PubMed Google Scholar
- J. Chudoba
You can also search for this author in PubMed Google Scholar
- J. J. Chwastowski
You can also search for this author in PubMed Google Scholar
- L. Chytka
You can also search for this author in PubMed Google Scholar
- D. Cieri
You can also search for this author in PubMed Google Scholar
- K. M. Ciesla
You can also search for this author in PubMed Google Scholar
- V. Cindro
You can also search for this author in PubMed Google Scholar
- I. A. Cioară
You can also search for this author in PubMed Google Scholar
- A. Ciocio
You can also search for this author in PubMed Google Scholar
- F. Cirotto
You can also search for this author in PubMed Google Scholar
- Z. H. Citron
You can also search for this author in PubMed Google Scholar
- M. Citterio
You can also search for this author in PubMed Google Scholar
- D. A. Ciubotaru
You can also search for this author in PubMed Google Scholar
- B. M. Ciungu
You can also search for this author in PubMed Google Scholar
- A. Clark
You can also search for this author in PubMed Google Scholar
- P. J. Clark
You can also search for this author in PubMed Google Scholar
- S. E. Clawson
You can also search for this author in PubMed Google Scholar
- C. Clement
You can also search for this author in PubMed Google Scholar
- L. Clissa
You can also search for this author in PubMed Google Scholar
- Y. Coadou
You can also search for this author in PubMed Google Scholar
- M. Cobal
You can also search for this author in PubMed Google Scholar
- A. Coccaro
You can also search for this author in PubMed Google Scholar
- J. Cochran
You can also search for this author in PubMed Google Scholar
- R. Coelho Lopes De Sa
You can also search for this author in PubMed Google Scholar
- H. Cohen
You can also search for this author in PubMed Google Scholar
- A. E. C. Coimbra
You can also search for this author in PubMed Google Scholar
- B. Cole
You can also search for this author in PubMed Google Scholar
- A. P. Colijn
You can also search for this author in PubMed Google Scholar
- J. Collot
You can also search for this author in PubMed Google Scholar
- P. Conde Muiño
You can also search for this author in PubMed Google Scholar
- S. H. Connell
You can also search for this author in PubMed Google Scholar
- I. A. Connelly
You can also search for this author in PubMed Google Scholar
- S. Constantinescu
You can also search for this author in PubMed Google Scholar
- F. Conventi
You can also search for this author in PubMed Google Scholar
- A. M. Cooper-Sarkar
You can also search for this author in PubMed Google Scholar
- F. Cormier
You can also search for this author in PubMed Google Scholar
- K. J. R. Cormier
You can also search for this author in PubMed Google Scholar
- L. D. Corpe
You can also search for this author in PubMed Google Scholar
- M. Corradi
You can also search for this author in PubMed Google Scholar
- E. E. Corrigan
You can also search for this author in PubMed Google Scholar
- F. Corriveau
You can also search for this author in PubMed Google Scholar
- M. J. Costa
You can also search for this author in PubMed Google Scholar
- F. Costanza
You can also search for this author in PubMed Google Scholar
- D. Costanzo
You can also search for this author in PubMed Google Scholar
- G. Cowan
You can also search for this author in PubMed Google Scholar
- J. W. Cowley
You can also search for this author in PubMed Google Scholar
- J. Crane
You can also search for this author in PubMed Google Scholar
- K. Cranmer
You can also search for this author in PubMed Google Scholar
- R. A. Creager
You can also search for this author in PubMed Google Scholar
- S. Crépé-Renaudin
You can also search for this author in PubMed Google Scholar
- F. Crescioli
You can also search for this author in PubMed Google Scholar
- M. Cristinziani
You can also search for this author in PubMed Google Scholar
- V. Croft
You can also search for this author in PubMed Google Scholar
- G. Crosetti
You can also search for this author in PubMed Google Scholar
- A. Cueto
You can also search for this author in PubMed Google Scholar
- T. Cuhadar Donszelmann
You can also search for this author in PubMed Google Scholar
- H. Cui
You can also search for this author in PubMed Google Scholar
- A. R. Cukierman
You can also search for this author in PubMed Google Scholar
- W. R. Cunningham
You can also search for this author in PubMed Google Scholar
- S. Czekierda
You can also search for this author in PubMed Google Scholar
- P. Czodrowski
You can also search for this author in PubMed Google Scholar
- M. M. Czurylo
You can also search for this author in PubMed Google Scholar
- M. J. Da Cunha Sargedas De Sousa
You can also search for this author in PubMed Google Scholar
- J. V. Da Fonseca Pinto
You can also search for this author in PubMed Google Scholar
- C. Da Via
You can also search for this author in PubMed Google Scholar
- W. Dabrowski
You can also search for this author in PubMed Google Scholar
- F. Dachs
You can also search for this author in PubMed Google Scholar
- T. Dado
You can also search for this author in PubMed Google Scholar
- S. Dahbi
You can also search for this author in PubMed Google Scholar
- T. Dai
You can also search for this author in PubMed Google Scholar
- C. Dallapiccola
You can also search for this author in PubMed Google Scholar
- M. Dam
You can also search for this author in PubMed Google Scholar
- G. D’amen
You can also search for this author in PubMed Google Scholar
- V. D’Amico
You can also search for this author in PubMed Google Scholar
- J. Damp
You can also search for this author in PubMed Google Scholar
- J. R. Dandoy
You can also search for this author in PubMed Google Scholar
- M. F. Daneri
You can also search for this author in PubMed Google Scholar
- M. Danninger
You can also search for this author in PubMed Google Scholar
- V. Dao
You can also search for this author in PubMed Google Scholar
- G. Darbo
You can also search for this author in PubMed Google Scholar
- O. Dartsi
You can also search for this author in PubMed Google Scholar
- A. Dattagupta
You can also search for this author in PubMed Google Scholar
- T. Daubney
You can also search for this author in PubMed Google Scholar
- S. D’Auria
You can also search for this author in PubMed Google Scholar
- C. David
You can also search for this author in PubMed Google Scholar
- T. Davidek
You can also search for this author in PubMed Google Scholar
- D. R. Davis
You can also search for this author in PubMed Google Scholar
- I. Dawson
You can also search for this author in PubMed Google Scholar
- K. De
You can also search for this author in PubMed Google Scholar
- R. De Asmundis
You can also search for this author in PubMed Google Scholar
- M. De Beurs
You can also search for this author in PubMed Google Scholar
- S. De Castro
You can also search for this author in PubMed Google Scholar
- N. De Groot
You can also search for this author in PubMed Google Scholar
- P. de Jong
You can also search for this author in PubMed Google Scholar
- H. De la Torre
You can also search for this author in PubMed Google Scholar
- A. De Maria
You can also search for this author in PubMed Google Scholar
- D. De Pedis
You can also search for this author in PubMed Google Scholar
- A. De Salvo
You can also search for this author in PubMed Google Scholar
- U. De Sanctis
You can also search for this author in PubMed Google Scholar
- M. De Santis
You can also search for this author in PubMed Google Scholar
- A. De Santo
You can also search for this author in PubMed Google Scholar
- J. B. De Vivie De Regie
You can also search for this author in PubMed Google Scholar
- D. V. Dedovich
You can also search for this author in PubMed Google Scholar
- A. M. Deiana
You can also search for this author in PubMed Google Scholar
- J. Del Peso
You can also search for this author in PubMed Google Scholar
- Y. Delabat Diaz
You can also search for this author in PubMed Google Scholar
- D. Delgove
You can also search for this author in PubMed Google Scholar
- F. Deliot
You can also search for this author in PubMed Google Scholar
- C. M. Delitzsch
You can also search for this author in PubMed Google Scholar
- M. Della Pietra
You can also search for this author in PubMed Google Scholar
- D. Della Volpe
You can also search for this author in PubMed Google Scholar
- A. Dell’Acqua
You can also search for this author in PubMed Google Scholar
- L. Dell’Asta
You can also search for this author in PubMed Google Scholar
- M. Delmastro
You can also search for this author in PubMed Google Scholar
- C. Delporte
You can also search for this author in PubMed Google Scholar
- P. A. Delsart
You can also search for this author in PubMed Google Scholar
- S. Demers
You can also search for this author in PubMed Google Scholar
- M. Demichev
You can also search for this author in PubMed Google Scholar
- G. Demontigny
You can also search for this author in PubMed Google Scholar
- S. P. Denisov
You can also search for this author in PubMed Google Scholar
- L. D’Eramo
You can also search for this author in PubMed Google Scholar
- D. Derendarz
You can also search for this author in PubMed Google Scholar
- J. E. Derkaoui
You can also search for this author in PubMed Google Scholar
- F. Derue
You can also search for this author in PubMed Google Scholar
- P. Dervan
You can also search for this author in PubMed Google Scholar
- K. Desch
You can also search for this author in PubMed Google Scholar
- K. Dette
You can also search for this author in PubMed Google Scholar
- C. Deutsch
You can also search for this author in PubMed Google Scholar
- M. R. Devesa
You can also search for this author in PubMed Google Scholar
- P. O. Deviveiros
You can also search for this author in PubMed Google Scholar
- F. A. Di Bello
You can also search for this author in PubMed Google Scholar
- A. Di Ciaccio
You can also search for this author in PubMed Google Scholar
- L. Di Ciaccio
You can also search for this author in PubMed Google Scholar
- C. Di Donato
You can also search for this author in PubMed Google Scholar
- A. Di Girolamo
You can also search for this author in PubMed Google Scholar
- G. Di Gregorio
You can also search for this author in PubMed Google Scholar
- A. Di Luca
You can also search for this author in PubMed Google Scholar
- B. Di Micco
You can also search for this author in PubMed Google Scholar
- R. Di Nardo
You can also search for this author in PubMed Google Scholar
- K. F. Di Petrillo
You can also search for this author in PubMed Google Scholar
- R. Di Sipio
You can also search for this author in PubMed Google Scholar
- C. Diaconu
You can also search for this author in PubMed Google Scholar
- F. A. Dias
You can also search for this author in PubMed Google Scholar
- T. Dias Do Vale
You can also search for this author in PubMed Google Scholar
- M. A. Diaz
You can also search for this author in PubMed Google Scholar
- F. G. Diaz Capriles
You can also search for this author in PubMed Google Scholar
- J. Dickinson
You can also search for this author in PubMed Google Scholar
- M. Didenko
You can also search for this author in PubMed Google Scholar
- E. B. Diehl
You can also search for this author in PubMed Google Scholar
- J. Dietrich
You can also search for this author in PubMed Google Scholar
- S. Díez Cornell
You can also search for this author in PubMed Google Scholar
- C. Diez Pardos
You can also search for this author in PubMed Google Scholar
- A. Dimitrievska
You can also search for this author in PubMed Google Scholar
- W. Ding
You can also search for this author in PubMed Google Scholar
- J. Dingfelder
You can also search for this author in PubMed Google Scholar
- S. J. Dittmeier
You can also search for this author in PubMed Google Scholar
- F. Dittus
You can also search for this author in PubMed Google Scholar
- F. Djama
You can also search for this author in PubMed Google Scholar
- T. Djobava
You can also search for this author in PubMed Google Scholar
- J. I. Djuvsland
You can also search for this author in PubMed Google Scholar
- M. A. B. Do Vale
You can also search for this author in PubMed Google Scholar
- M. Dobre
You can also search for this author in PubMed Google Scholar
- D. Dodsworth
You can also search for this author in PubMed Google Scholar
- C. Doglioni
You can also search for this author in PubMed Google Scholar
- J. Dolejsi
You can also search for this author in PubMed Google Scholar
- Z. Dolezal
You can also search for this author in PubMed Google Scholar
- M. Donadelli
You can also search for this author in PubMed Google Scholar
- B. Dong
You can also search for this author in PubMed Google Scholar
- J. Donini
You can also search for this author in PubMed Google Scholar
- A. D’onofrio
You can also search for this author in PubMed Google Scholar
- M. D’Onofrio
You can also search for this author in PubMed Google Scholar
- J. Dopke
You can also search for this author in PubMed Google Scholar
- A. Doria
You can also search for this author in PubMed Google Scholar
- M. T. Dova
You can also search for this author in PubMed Google Scholar
- A. T. Doyle
You can also search for this author in PubMed Google Scholar
- E. Drechsler
You can also search for this author in PubMed Google Scholar
- E. Dreyer
You can also search for this author in PubMed Google Scholar
- T. Dreyer
You can also search for this author in PubMed Google Scholar
- A. S. Drobac
You can also search for this author in PubMed Google Scholar
- D. Du
You can also search for this author in PubMed Google Scholar
- T. A. du Pree
You can also search for this author in PubMed Google Scholar
- Y. Duan
You can also search for this author in PubMed Google Scholar
- F. Dubinin
You can also search for this author in PubMed Google Scholar
- M. Dubovsky
You can also search for this author in PubMed Google Scholar
- A. Dubreuil
You can also search for this author in PubMed Google Scholar
- E. Duchovni
You can also search for this author in PubMed Google Scholar
- G. Duckeck
You can also search for this author in PubMed Google Scholar
- O. A. Ducu
You can also search for this author in PubMed Google Scholar
- D. Duda
You can also search for this author in PubMed Google Scholar
- A. Dudarev
You can also search for this author in PubMed Google Scholar
- A. C. Dudder
You can also search for this author in PubMed Google Scholar
- E. M. Duffield
You can also search for this author in PubMed Google Scholar
- M. D’uffizi
You can also search for this author in PubMed Google Scholar
- L. Duflot
You can also search for this author in PubMed Google Scholar
- M. Dührssen
You can also search for this author in PubMed Google Scholar
- C. Dülsen
You can also search for this author in PubMed Google Scholar
- M. Dumancic
You can also search for this author in PubMed Google Scholar
- A. E. Dumitriu
You can also search for this author in PubMed Google Scholar
- M. Dunford
You can also search for this author in PubMed Google Scholar
- S. Dungs
You can also search for this author in PubMed Google Scholar
- A. Duperrin
You can also search for this author in PubMed Google Scholar
- H. Duran Yildiz
You can also search for this author in PubMed Google Scholar
- M. Düren
You can also search for this author in PubMed Google Scholar
- A. Durglishvili
You can also search for this author in PubMed Google Scholar
- D. Duschinger
You can also search for this author in PubMed Google Scholar
- B. Dutta
You can also search for this author in PubMed Google Scholar
- D. Duvnjak
You can also search for this author in PubMed Google Scholar
- G. I. Dyckes
You can also search for this author in PubMed Google Scholar
- M. Dyndal
You can also search for this author in PubMed Google Scholar
- S. Dysch
You can also search for this author in PubMed Google Scholar
- B. S. Dziedzic
You can also search for this author in PubMed Google Scholar
- M. G. Eggleston
You can also search for this author in PubMed Google Scholar
- T. Eifert
You can also search for this author in PubMed Google Scholar
- G. Eigen
You can also search for this author in PubMed Google Scholar
- K. Einsweiler
You can also search for this author in PubMed Google Scholar
- T. Ekelof
You can also search for this author in PubMed Google Scholar
- H. El Jarrari
You can also search for this author in PubMed Google Scholar
- V. Ellajosyula
You can also search for this author in PubMed Google Scholar
- M. Ellert
You can also search for this author in PubMed Google Scholar
- F. Ellinghaus
You can also search for this author in PubMed Google Scholar
- A. A. Elliot
You can also search for this author in PubMed Google Scholar
- N. Ellis
You can also search for this author in PubMed Google Scholar
- J. Elmsheuser
You can also search for this author in PubMed Google Scholar
- M. Elsing
You can also search for this author in PubMed Google Scholar
- D. Emeliyanov
You can also search for this author in PubMed Google Scholar
- A. Emerman
You can also search for this author in PubMed Google Scholar
- Y. Enari
You can also search for this author in PubMed Google Scholar
- M. B. Epland
You can also search for this author in PubMed Google Scholar
- J. Erdmann
You can also search for this author in PubMed Google Scholar
- A. Ereditato
You can also search for this author in PubMed Google Scholar
- P. A. Erland
You can also search for this author in PubMed Google Scholar
- M. Errenst
You can also search for this author in PubMed Google Scholar
- M. Escalier
You can also search for this author in PubMed Google Scholar
- C. Escobar
You can also search for this author in PubMed Google Scholar
- O. Estrada Pastor
You can also search for this author in PubMed Google Scholar
- E. Etzion
You can also search for this author in PubMed Google Scholar
- G. Evans
You can also search for this author in PubMed Google Scholar
- H. Evans
You can also search for this author in PubMed Google Scholar
- M. O. Evans
You can also search for this author in PubMed Google Scholar
- A. Ezhilov
You can also search for this author in PubMed Google Scholar
- F. Fabbri
You can also search for this author in PubMed Google Scholar
- L. Fabbri
You can also search for this author in PubMed Google Scholar
- V. Fabiani
You can also search for this author in PubMed Google Scholar
- G. Facini
You can also search for this author in PubMed Google Scholar
- R. M. Fakhrutdinov
You can also search for this author in PubMed Google Scholar
- S. Falciano
You can also search for this author in PubMed Google Scholar
- P. J. Falke
You can also search for this author in PubMed Google Scholar
- S. Falke
You can also search for this author in PubMed Google Scholar
- J. Faltova
You can also search for this author in PubMed Google Scholar
- Y. Fang
You can also search for this author in PubMed Google Scholar
- Y. Fang
You can also search for this author in PubMed Google Scholar
- G. Fanourakis
You can also search for this author in PubMed Google Scholar
- M. Fanti
You can also search for this author in PubMed Google Scholar
- M. Faraj
You can also search for this author in PubMed Google Scholar
- A. Farbin
You can also search for this author in PubMed Google Scholar
- A. Farilla
You can also search for this author in PubMed Google Scholar
- E. M. Farina
You can also search for this author in PubMed Google Scholar
- T. Farooque
You can also search for this author in PubMed Google Scholar
- S. M. Farrington
You can also search for this author in PubMed Google Scholar
- P. Farthouat
You can also search for this author in PubMed Google Scholar
- F. Fassi
You can also search for this author in PubMed Google Scholar
- P. Fassnacht
You can also search for this author in PubMed Google Scholar
- D. Fassouliotis
You can also search for this author in PubMed Google Scholar
- M. Faucci Giannelli
You can also search for this author in PubMed Google Scholar
- W. J. Fawcett
You can also search for this author in PubMed Google Scholar
- L. Fayard
You can also search for this author in PubMed Google Scholar
- O. L. Fedin
You can also search for this author in PubMed Google Scholar
- W. Fedorko
You can also search for this author in PubMed Google Scholar
- A. Fehr
You can also search for this author in PubMed Google Scholar
- M. Feickert
You can also search for this author in PubMed Google Scholar
- L. Feligioni
You can also search for this author in PubMed Google Scholar
- A. Fell
You can also search for this author in PubMed Google Scholar
- C. Feng
You can also search for this author in PubMed Google Scholar
- M. Feng
You can also search for this author in PubMed Google Scholar
- M. J. Fenton
You can also search for this author in PubMed Google Scholar
- A. B. Fenyuk
You can also search for this author in PubMed Google Scholar
- S. W. Ferguson
You can also search for this author in PubMed Google Scholar
- J. Ferrando
You can also search for this author in PubMed Google Scholar
- A. Ferrari
You can also search for this author in PubMed Google Scholar
- P. Ferrari
You can also search for this author in PubMed Google Scholar
- R. Ferrari
You can also search for this author in PubMed Google Scholar
- D. E. Ferreira de Lima
You can also search for this author in PubMed Google Scholar
- A. Ferrer
You can also search for this author in PubMed Google Scholar
- D. Ferrere
You can also search for this author in PubMed Google Scholar
- C. Ferretti
You can also search for this author in PubMed Google Scholar
- F. Fiedler
You can also search for this author in PubMed Google Scholar
- A. Filipčič
You can also search for this author in PubMed Google Scholar
- F. Filthaut
You can also search for this author in PubMed Google Scholar
- K. D. Finelli
You can also search for this author in PubMed Google Scholar
- M. C. N. Fiolhais
You can also search for this author in PubMed Google Scholar
- L. Fiorini
You can also search for this author in PubMed Google Scholar
- F. Fischer
You can also search for this author in PubMed Google Scholar
- J. Fischer
You can also search for this author in PubMed Google Scholar
- W. C. Fisher
You can also search for this author in PubMed Google Scholar
- T. Fitschen
You can also search for this author in PubMed Google Scholar
- I. Fleck
You can also search for this author in PubMed Google Scholar
- P. Fleischmann
You can also search for this author in PubMed Google Scholar
- T. Flick
You can also search for this author in PubMed Google Scholar
- B. M. Flierl
You can also search for this author in PubMed Google Scholar
- L. Flores
You can also search for this author in PubMed Google Scholar
- L. R. Flores Castillo
You can also search for this author in PubMed Google Scholar
- F. M. Follega
You can also search for this author in PubMed Google Scholar
- N. Fomin
You can also search for this author in PubMed Google Scholar
- J. H. Foo
You can also search for this author in PubMed Google Scholar
- G. T. Forcolin
You can also search for this author in PubMed Google Scholar
- B. C. Forland
You can also search for this author in PubMed Google Scholar
- A. Formica
You can also search for this author in PubMed Google Scholar
- F. A. Förster
You can also search for this author in PubMed Google Scholar
- A. C. Forti
You can also search for this author in PubMed Google Scholar
- E. Fortin
You can also search for this author in PubMed Google Scholar
- M. G. Foti
You can also search for this author in PubMed Google Scholar
- D. Fournier
You can also search for this author in PubMed Google Scholar
- H. Fox
You can also search for this author in PubMed Google Scholar
- P. Francavilla
You can also search for this author in PubMed Google Scholar
- S. Francescato
You can also search for this author in PubMed Google Scholar
- M. Franchini
You can also search for this author in PubMed Google Scholar
- S. Franchino
You can also search for this author in PubMed Google Scholar
- D. Francis
You can also search for this author in PubMed Google Scholar
- L. Franco
You can also search for this author in PubMed Google Scholar
- L. Franconi
You can also search for this author in PubMed Google Scholar
- M. Franklin
You can also search for this author in PubMed Google Scholar
- G. Frattari
You can also search for this author in PubMed Google Scholar
- A. N. Fray
You can also search for this author in PubMed Google Scholar
- P. M. Freeman
You can also search for this author in PubMed Google Scholar
- B. Freund
You can also search for this author in PubMed Google Scholar
- W. S. Freund
You can also search for this author in PubMed Google Scholar
- E. M. Freundlich
You can also search for this author in PubMed Google Scholar
- D. C. Frizzell
You can also search for this author in PubMed Google Scholar
- D. Froidevaux
You can also search for this author in PubMed Google Scholar
- J. A. Frost
You can also search for this author in PubMed Google Scholar
- M. Fujimoto
You can also search for this author in PubMed Google Scholar
- C. Fukunaga
You can also search for this author in PubMed Google Scholar
- E. Fullana Torregrosa
You can also search for this author in PubMed Google Scholar
- T. Fusayasu
You can also search for this author in PubMed Google Scholar
- J. Fuster
You can also search for this author in PubMed Google Scholar
- A. Gabrielli
You can also search for this author in PubMed Google Scholar
- A. Gabrielli
You can also search for this author in PubMed Google Scholar
- S. Gadatsch
You can also search for this author in PubMed Google Scholar
- P. Gadow
You can also search for this author in PubMed Google Scholar
- G. Gagliardi
You can also search for this author in PubMed Google Scholar
- L. G. Gagnon
You can also search for this author in PubMed Google Scholar
- G. E. Gallardo
You can also search for this author in PubMed Google Scholar
- E. J. Gallas
You can also search for this author in PubMed Google Scholar
- B. J. Gallop
You can also search for this author in PubMed Google Scholar
- R. Gamboa Goni
You can also search for this author in PubMed Google Scholar
- K. K. Gan
You can also search for this author in PubMed Google Scholar
- S. Ganguly
You can also search for this author in PubMed Google Scholar
- J. Gao
You can also search for this author in PubMed Google Scholar
- Y. Gao
You can also search for this author in PubMed Google Scholar
- Y. S. Gao
You can also search for this author in PubMed Google Scholar
- F. M. Garay Walls
You can also search for this author in PubMed Google Scholar
- C. García
You can also search for this author in PubMed Google Scholar
- J. E. García Navarro
You can also search for this author in PubMed Google Scholar
- J. A. García Pascual
You can also search for this author in PubMed Google Scholar
- C. Garcia-Argos
You can also search for this author in PubMed Google Scholar
- M. Garcia-Sciveres
You can also search for this author in PubMed Google Scholar
- R. W. Gardner
You can also search for this author in PubMed Google Scholar
- N. Garelli
You can also search for this author in PubMed Google Scholar
- S. Gargiulo
You can also search for this author in PubMed Google Scholar
- C. A. Garner
You can also search for this author in PubMed Google Scholar
- V. Garonne
You can also search for this author in PubMed Google Scholar
- S. J. Gasiorowski
You can also search for this author in PubMed Google Scholar
- P. Gaspar
You can also search for this author in PubMed Google Scholar
- A. Gaudiello
You can also search for this author in PubMed Google Scholar
- G. Gaudio
You can also search for this author in PubMed Google Scholar
- P. Gauzzi
You can also search for this author in PubMed Google Scholar
- I. L. Gavrilenko
You can also search for this author in PubMed Google Scholar
- A. Gavrilyuk
You can also search for this author in PubMed Google Scholar
- C. Gay
You can also search for this author in PubMed Google Scholar
- G. Gaycken
You can also search for this author in PubMed Google Scholar
- E. N. Gazis
You can also search for this author in PubMed Google Scholar
- A. A. Geanta
You can also search for this author in PubMed Google Scholar
- C. M. Gee
You can also search for this author in PubMed Google Scholar
- C. N. P. Gee
You can also search for this author in PubMed Google Scholar
- J. Geisen
You can also search for this author in PubMed Google Scholar
- M. Geisen
You can also search for this author in PubMed Google Scholar
- C. Gemme
You can also search for this author in PubMed Google Scholar
- M. H. Genest
You can also search for this author in PubMed Google Scholar
- C. Geng
You can also search for this author in PubMed Google Scholar
- S. Gentile
You can also search for this author in PubMed Google Scholar
- S. George
You can also search for this author in PubMed Google Scholar
- T. Geralis
You can also search for this author in PubMed Google Scholar
- L. O. Gerlach
You can also search for this author in PubMed Google Scholar
- P. Gessinger-Befurt
You can also search for this author in PubMed Google Scholar
- G. Gessner
You can also search for this author in PubMed Google Scholar
- M. Ghasemi Bostanabad
You can also search for this author in PubMed Google Scholar
- M. Ghneimat
You can also search for this author in PubMed Google Scholar
- A. Ghosh
You can also search for this author in PubMed Google Scholar
- A. Ghosh
You can also search for this author in PubMed Google Scholar
- B. Giacobbe
You can also search for this author in PubMed Google Scholar
- S. Giagu
You can also search for this author in PubMed Google Scholar
- N. Giangiacomi
You can also search for this author in PubMed Google Scholar
- P. Giannetti
You can also search for this author in PubMed Google Scholar
- A. Giannini
You can also search for this author in PubMed Google Scholar
- G. Giannini
You can also search for this author in PubMed Google Scholar
- S. M. Gibson
You can also search for this author in PubMed Google Scholar
- M. Gignac
You can also search for this author in PubMed Google Scholar
- D. T. Gil
You can also search for this author in PubMed Google Scholar
- B. J. Gilbert
You can also search for this author in PubMed Google Scholar
- D. Gillberg
You can also search for this author in PubMed Google Scholar
- G. Gilles
You can also search for this author in PubMed Google Scholar
- N. E. K. Gillwald
You can also search for this author in PubMed Google Scholar
- D. M. Gingrich
You can also search for this author in PubMed Google Scholar
- M. P. Giordani
You can also search for this author in PubMed Google Scholar
- P. F. Giraud
You can also search for this author in PubMed Google Scholar
- G. Giugliarelli
You can also search for this author in PubMed Google Scholar
- D. Giugni
You can also search for this author in PubMed Google Scholar
- F. Giuli
You can also search for this author in PubMed Google Scholar
- S. Gkaitatzis
You can also search for this author in PubMed Google Scholar
- I. Gkialas
You can also search for this author in PubMed Google Scholar
- E. L. Gkougkousis
You can also search for this author in PubMed Google Scholar
- P. Gkountoumis
You can also search for this author in PubMed Google Scholar
- L. K. Gladilin
You can also search for this author in PubMed Google Scholar
- C. Glasman
You can also search for this author in PubMed Google Scholar
- J. Glatzer
You can also search for this author in PubMed Google Scholar
- P. C. F. Glaysher
You can also search for this author in PubMed Google Scholar
- A. Glazov
You can also search for this author in PubMed Google Scholar
- G. R. Gledhill
You can also search for this author in PubMed Google Scholar
- I. Gnesi
You can also search for this author in PubMed Google Scholar
- M. Goblirsch-Kolb
You can also search for this author in PubMed Google Scholar
- D. Godin
You can also search for this author in PubMed Google Scholar
- S. Goldfarb
You can also search for this author in PubMed Google Scholar
- T. Golling
You can also search for this author in PubMed Google Scholar
- D. Golubkov
You can also search for this author in PubMed Google Scholar
- A. Gomes
You can also search for this author in PubMed Google Scholar
- R. Goncalves Gama
You can also search for this author in PubMed Google Scholar
- R. Gonçalo
You can also search for this author in PubMed Google Scholar
- G. Gonella
You can also search for this author in PubMed Google Scholar
- L. Gonella
You can also search for this author in PubMed Google Scholar
- A. Gongadze
You can also search for this author in PubMed Google Scholar
- F. Gonnella
You can also search for this author in PubMed Google Scholar
- J. L. Gonski
You can also search for this author in PubMed Google Scholar
- S. González de la Hoz
You can also search for this author in PubMed Google Scholar
- S. Gonzalez Fernandez
You can also search for this author in PubMed Google Scholar
- R. Gonzalez Lopez
You can also search for this author in PubMed Google Scholar
- C. Gonzalez Renteria
You can also search for this author in PubMed Google Scholar
- R. Gonzalez Suarez
You can also search for this author in PubMed Google Scholar
- S. Gonzalez-Sevilla
You can also search for this author in PubMed Google Scholar
- G. R. Gonzalvo Rodriguez
You can also search for this author in PubMed Google Scholar
- L. Goossens
You can also search for this author in PubMed Google Scholar
- N. A. Gorasia
You can also search for this author in PubMed Google Scholar
- P. A. Gorbounov
You can also search for this author in PubMed Google Scholar
- H. A. Gordon
You can also search for this author in PubMed Google Scholar
- B. Gorini
You can also search for this author in PubMed Google Scholar
- E. Gorini
You can also search for this author in PubMed Google Scholar
- A. Gorišek
You can also search for this author in PubMed Google Scholar
- A. T. Goshaw
You can also search for this author in PubMed Google Scholar
- M. I. Gostkin
You can also search for this author in PubMed Google Scholar
- C. A. Gottardo
You can also search for this author in PubMed Google Scholar
- M. Gouighri
You can also search for this author in PubMed Google Scholar
- A. G. Goussiou
You can also search for this author in PubMed Google Scholar
- N. Govender
You can also search for this author in PubMed Google Scholar
- C. Goy
You can also search for this author in PubMed Google Scholar
- I. Grabowska-Bold
You can also search for this author in PubMed Google Scholar
- E. C. Graham
You can also search for this author in PubMed Google Scholar
- J. Gramling
You can also search for this author in PubMed Google Scholar
- E. Gramstad
You can also search for this author in PubMed Google Scholar
- S. Grancagnolo
You can also search for this author in PubMed Google Scholar
- M. Grandi
You can also search for this author in PubMed Google Scholar
- V. Gratchev
You can also search for this author in PubMed Google Scholar
- P. M. Gravila
You can also search for this author in PubMed Google Scholar
- F. G. Gravili
You can also search for this author in PubMed Google Scholar
- C. Gray
You can also search for this author in PubMed Google Scholar
- H. M. Gray
You can also search for this author in PubMed Google Scholar
- C. Grefe
You can also search for this author in PubMed Google Scholar
- K. Gregersen
You can also search for this author in PubMed Google Scholar
- I. M. Gregor
You can also search for this author in PubMed Google Scholar
- P. Grenier
You can also search for this author in PubMed Google Scholar
- K. Grevtsov
You can also search for this author in PubMed Google Scholar
- C. Grieco
You can also search for this author in PubMed Google Scholar
- N. A. Grieser
You can also search for this author in PubMed Google Scholar
- A. A. Grillo
You can also search for this author in PubMed Google Scholar
- K. Grimm
You can also search for this author in PubMed Google Scholar
- S. Grinstein
You can also search for this author in PubMed Google Scholar
- J.-F. Grivaz
You can also search for this author in PubMed Google Scholar
- S. Groh
You can also search for this author in PubMed Google Scholar
- E. Gross
You can also search for this author in PubMed Google Scholar
- J. Grosse-Knetter
You can also search for this author in PubMed Google Scholar
- Z. J. Grout
You can also search for this author in PubMed Google Scholar
- C. Grud
You can also search for this author in PubMed Google Scholar
- A. Grummer
You can also search for this author in PubMed Google Scholar
- J. C. Grundy
You can also search for this author in PubMed Google Scholar
- L. Guan
You can also search for this author in PubMed Google Scholar
- W. Guan
You can also search for this author in PubMed Google Scholar
- C. Gubbels
You can also search for this author in PubMed Google Scholar
- J. Guenther
You can also search for this author in PubMed Google Scholar
- A. Guerguichon
You can also search for this author in PubMed Google Scholar
- J. G. R. Guerrero Rojas
You can also search for this author in PubMed Google Scholar
- F. Guescini
You can also search for this author in PubMed Google Scholar
- D. Guest
You can also search for this author in PubMed Google Scholar
- R. Gugel
You can also search for this author in PubMed Google Scholar
- A. Guida
You can also search for this author in PubMed Google Scholar
- T. Guillemin
You can also search for this author in PubMed Google Scholar
- S. Guindon
You can also search for this author in PubMed Google Scholar
- J. Guo
You can also search for this author in PubMed Google Scholar
- W. Guo
You can also search for this author in PubMed Google Scholar
- Y. Guo
You can also search for this author in PubMed Google Scholar
- Z. Guo
You can also search for this author in PubMed Google Scholar
- R. Gupta
You can also search for this author in PubMed Google Scholar
- S. Gurbuz
You can also search for this author in PubMed Google Scholar
- G. Gustavino
You can also search for this author in PubMed Google Scholar
- M. Guth
You can also search for this author in PubMed Google Scholar
- P. Gutierrez
You can also search for this author in PubMed Google Scholar
- C. Gutschow
You can also search for this author in PubMed Google Scholar
- C. Guyot
You can also search for this author in PubMed Google Scholar
- C. Gwenlan
You can also search for this author in PubMed Google Scholar
- C. B. Gwilliam
You can also search for this author in PubMed Google Scholar
- E. S. Haaland
You can also search for this author in PubMed Google Scholar
- A. Haas
You can also search for this author in PubMed Google Scholar
- C. Haber
You can also search for this author in PubMed Google Scholar
- H. K. Hadavand
You can also search for this author in PubMed Google Scholar
- A. Hadef
You can also search for this author in PubMed Google Scholar
- M. Haleem
You can also search for this author in PubMed Google Scholar
- J. Haley
You can also search for this author in PubMed Google Scholar
- J. J. Hall
You can also search for this author in PubMed Google Scholar
- G. Halladjian
You can also search for this author in PubMed Google Scholar
- G. D. Hallewell
You can also search for this author in PubMed Google Scholar
- K. Hamano
You can also search for this author in PubMed Google Scholar
- H. Hamdaoui
You can also search for this author in PubMed Google Scholar
- M. Hamer
You can also search for this author in PubMed Google Scholar
- G. N. Hamity
You can also search for this author in PubMed Google Scholar
- K. Han
You can also search for this author in PubMed Google Scholar
- L. Han
You can also search for this author in PubMed Google Scholar
- L. Han
You can also search for this author in PubMed Google Scholar
- S. Han
You can also search for this author in PubMed Google Scholar
- Y. F. Han
You can also search for this author in PubMed Google Scholar
- K. Hanagaki
You can also search for this author in PubMed Google Scholar
- M. Hance
You can also search for this author in PubMed Google Scholar
- D. M. Handl
You can also search for this author in PubMed Google Scholar
- M. D. Hank
You can also search for this author in PubMed Google Scholar
- R. Hankache
You can also search for this author in PubMed Google Scholar
- E. Hansen
You can also search for this author in PubMed Google Scholar
- J. B. Hansen
You can also search for this author in PubMed Google Scholar
- J. D. Hansen
You can also search for this author in PubMed Google Scholar
- M. C. Hansen
You can also search for this author in PubMed Google Scholar
- P. H. Hansen
You can also search for this author in PubMed Google Scholar
- E. C. Hanson
You can also search for this author in PubMed Google Scholar
- K. Hara
You can also search for this author in PubMed Google Scholar
- T. Harenberg
You can also search for this author in PubMed Google Scholar
- S. Harkusha
You can also search for this author in PubMed Google Scholar
- P. F. Harrison
You can also search for this author in PubMed Google Scholar
- N. M. Hartman
You can also search for this author in PubMed Google Scholar
- N. M. Hartmann
You can also search for this author in PubMed Google Scholar
- Y. Hasegawa
You can also search for this author in PubMed Google Scholar
- A. Hasib
You can also search for this author in PubMed Google Scholar
- S. Hassani
You can also search for this author in PubMed Google Scholar
- S. Haug
You can also search for this author in PubMed Google Scholar
- R. Hauser
You can also search for this author in PubMed Google Scholar
- M. Havranek
You can also search for this author in PubMed Google Scholar
- C. M. Hawkes
You can also search for this author in PubMed Google Scholar
- R. J. Hawkings
You can also search for this author in PubMed Google Scholar
- S. Hayashida
You can also search for this author in PubMed Google Scholar
- D. Hayden
You can also search for this author in PubMed Google Scholar
- C. Hayes
You can also search for this author in PubMed Google Scholar
- R. L. Hayes
You can also search for this author in PubMed Google Scholar
- C. P. Hays
You can also search for this author in PubMed Google Scholar
- J. M. Hays
You can also search for this author in PubMed Google Scholar
- H. S. Hayward
You can also search for this author in PubMed Google Scholar
- S. J. Haywood
You can also search for this author in PubMed Google Scholar
- F. He
You can also search for this author in PubMed Google Scholar
- Y. He
You can also search for this author in PubMed Google Scholar
- M. P. Heath
You can also search for this author in PubMed Google Scholar
- V. Hedberg
You can also search for this author in PubMed Google Scholar
- A. L. Heggelund
You can also search for this author in PubMed Google Scholar
- N. D. Hehir
You can also search for this author in PubMed Google Scholar
- C. Heidegger
You can also search for this author in PubMed Google Scholar
- K. K. Heidegger
You can also search for this author in PubMed Google Scholar
- W. D. Heidorn
You can also search for this author in PubMed Google Scholar
- J. Heilman
You can also search for this author in PubMed Google Scholar
- S. Heim
You can also search for this author in PubMed Google Scholar
- T. Heim
You can also search for this author in PubMed Google Scholar
- B. Heinemann
You can also search for this author in PubMed Google Scholar
- J. G. Heinlein
You can also search for this author in PubMed Google Scholar
- J. J. Heinrich
You can also search for this author in PubMed Google Scholar
- L. Heinrich
You can also search for this author in PubMed Google Scholar
- J. Hejbal
You can also search for this author in PubMed Google Scholar
- L. Helary
You can also search for this author in PubMed Google Scholar
- A. Held
You can also search for this author in PubMed Google Scholar
- S. Hellesund
You can also search for this author in PubMed Google Scholar
- C. M. Helling
You can also search for this author in PubMed Google Scholar
- S. Hellman
You can also search for this author in PubMed Google Scholar
- C. Helsens
You can also search for this author in PubMed Google Scholar
- R. C. W. Henderson
You can also search for this author in PubMed Google Scholar
- L. Henkelmann
You can also search for this author in PubMed Google Scholar
- A. M. Henriques Correia
You can also search for this author in PubMed Google Scholar
- H. Herde
You can also search for this author in PubMed Google Scholar
- Y. Hernández Jiménez
You can also search for this author in PubMed Google Scholar
- H. Herr
You can also search for this author in PubMed Google Scholar
- M. G. Herrmann
You can also search for this author in PubMed Google Scholar
- T. Herrmann
You can also search for this author in PubMed Google Scholar
- G. Herten
You can also search for this author in PubMed Google Scholar
- R. Hertenberger
You can also search for this author in PubMed Google Scholar
- L. Hervas
You can also search for this author in PubMed Google Scholar
- G. G. Hesketh
You can also search for this author in PubMed Google Scholar
- N. P. Hessey
You can also search for this author in PubMed Google Scholar
- H. Hibi
You can also search for this author in PubMed Google Scholar
- S. Higashino
You can also search for this author in PubMed Google Scholar
- E. Higón-Rodriguez
You can also search for this author in PubMed Google Scholar
- K. Hildebrand
You can also search for this author in PubMed Google Scholar
- J. C. Hill
You can also search for this author in PubMed Google Scholar
- K. K. Hill
You can also search for this author in PubMed Google Scholar
- K. H. Hiller
You can also search for this author in PubMed Google Scholar
- S. J. Hillier
You can also search for this author in PubMed Google Scholar
- M. Hils
You can also search for this author in PubMed Google Scholar
- I. Hinchliffe
You can also search for this author in PubMed Google Scholar
- F. Hinterkeuser
You can also search for this author in PubMed Google Scholar
- M. Hirose
You can also search for this author in PubMed Google Scholar
- S. Hirose
You can also search for this author in PubMed Google Scholar
- D. Hirschbuehl
You can also search for this author in PubMed Google Scholar
- B. Hiti
You can also search for this author in PubMed Google Scholar
- O. Hladik
You can also search for this author in PubMed Google Scholar
- J. Hobbs
You can also search for this author in PubMed Google Scholar
- R. Hobincu
You can also search for this author in PubMed Google Scholar
- N. Hod
You can also search for this author in PubMed Google Scholar
- M. C. Hodgkinson
You can also search for this author in PubMed Google Scholar
- A. Hoecker
You can also search for this author in PubMed Google Scholar
- D. Hohn
You can also search for this author in PubMed Google Scholar
- D. Hohov
You can also search for this author in PubMed Google Scholar
- T. Holm
You can also search for this author in PubMed Google Scholar
- T. R. Holmes
You can also search for this author in PubMed Google Scholar
- M. Holzbock
You can also search for this author in PubMed Google Scholar
- L. B. A. H. Hommels
You can also search for this author in PubMed Google Scholar
- T. M. Hong
You can also search for this author in PubMed Google Scholar
- J. C. Honig
You can also search for this author in PubMed Google Scholar
- A. Hönle
You can also search for this author in PubMed Google Scholar
- B. H. Hooberman
You can also search for this author in PubMed Google Scholar
- W. H. Hopkins
You can also search for this author in PubMed Google Scholar
- Y. Horii
You can also search for this author in PubMed Google Scholar
- P. Horn
You can also search for this author in PubMed Google Scholar
- L. A. Horyn
You can also search for this author in PubMed Google Scholar
- S. Hou
You can also search for this author in PubMed Google Scholar
- A. Hoummada
You can also search for this author in PubMed Google Scholar
- J. Howarth
You can also search for this author in PubMed Google Scholar
- J. Hoya
You can also search for this author in PubMed Google Scholar
- M. Hrabovsky
You can also search for this author in PubMed Google Scholar
- J. Hrivnac
You can also search for this author in PubMed Google Scholar
- A. Hrynevich
You can also search for this author in PubMed Google Scholar
- T. Hryn’ova
You can also search for this author in PubMed Google Scholar
- P. J. Hsu
You can also search for this author in PubMed Google Scholar
- S.-C. Hsu
You can also search for this author in PubMed Google Scholar
- Q. Hu
You can also search for this author in PubMed Google Scholar
- S. Hu
You can also search for this author in PubMed Google Scholar
- Y. F. Hu
You can also search for this author in PubMed Google Scholar
- D. P. Huang
You can also search for this author in PubMed Google Scholar
- X. Huang
You can also search for this author in PubMed Google Scholar
- Y. Huang
You can also search for this author in PubMed Google Scholar
- Y. Huang
You can also search for this author in PubMed Google Scholar
- Z. Hubacek
You can also search for this author in PubMed Google Scholar
- F. Hubaut
You can also search for this author in PubMed Google Scholar
- M. Huebner
You can also search for this author in PubMed Google Scholar
- F. Huegging
You can also search for this author in PubMed Google Scholar
- T. B. Huffman
You can also search for this author in PubMed Google Scholar
- M. Huhtinen
You can also search for this author in PubMed Google Scholar
- R. Hulsken
You can also search for this author in PubMed Google Scholar
- R. F. H. Hunter
You can also search for this author in PubMed Google Scholar
- N. Huseynov
You can also search for this author in PubMed Google Scholar
- J. Huston
You can also search for this author in PubMed Google Scholar
- J. Huth
You can also search for this author in PubMed Google Scholar
- R. Hyneman
You can also search for this author in PubMed Google Scholar
- S. Hyrych
You can also search for this author in PubMed Google Scholar
- G. Iacobucci
You can also search for this author in PubMed Google Scholar
- G. Iakovidis
You can also search for this author in PubMed Google Scholar
- I. Ibragimov
You can also search for this author in PubMed Google Scholar
- L. Iconomidou-Fayard
You can also search for this author in PubMed Google Scholar
- P. Iengo
You can also search for this author in PubMed Google Scholar
- R. Ignazzi
You can also search for this author in PubMed Google Scholar
- R. Iguchi
You can also search for this author in PubMed Google Scholar
- T. Iizawa
You can also search for this author in PubMed Google Scholar
- Y. Ikegami
You can also search for this author in PubMed Google Scholar
- M. Ikeno
You can also search for this author in PubMed Google Scholar
- N. Ilic
You can also search for this author in PubMed Google Scholar
- F. Iltzsche
You can also search for this author in PubMed Google Scholar
- H. Imam
You can also search for this author in PubMed Google Scholar
- G. Introzzi
You can also search for this author in PubMed Google Scholar
- M. Iodice
You can also search for this author in PubMed Google Scholar
- K. Iordanidou
You can also search for this author in PubMed Google Scholar
- V. Ippolito
You can also search for this author in PubMed Google Scholar
- M. F. Isacson
You can also search for this author in PubMed Google Scholar
- M. Ishino
You can also search for this author in PubMed Google Scholar
- W. Islam
You can also search for this author in PubMed Google Scholar
- C. Issever
You can also search for this author in PubMed Google Scholar
- S. Istin
You can also search for this author in PubMed Google Scholar
- J. M. Iturbe Ponce
You can also search for this author in PubMed Google Scholar
- R. Iuppa
You can also search for this author in PubMed Google Scholar
- A. Ivina
You can also search for this author in PubMed Google Scholar
- J. M. Izen
You can also search for this author in PubMed Google Scholar
- V. Izzo
You can also search for this author in PubMed Google Scholar
- P. Jacka
You can also search for this author in PubMed Google Scholar
- P. Jackson
You can also search for this author in PubMed Google Scholar
- R. M. Jacobs
You can also search for this author in PubMed Google Scholar
- B. P. Jaeger
You can also search for this author in PubMed Google Scholar
- V. Jain
You can also search for this author in PubMed Google Scholar
- G. Jäkel
You can also search for this author in PubMed Google Scholar
- K. B. Jakobi
You can also search for this author in PubMed Google Scholar
- K. Jakobs
You can also search for this author in PubMed Google Scholar
- T. Jakoubek
You can also search for this author in PubMed Google Scholar
- J. Jamieson
You can also search for this author in PubMed Google Scholar
- K. W. Janas
You can also search for this author in PubMed Google Scholar
- R. Jansky
You can also search for this author in PubMed Google Scholar
- M. Janus
You can also search for this author in PubMed Google Scholar
- P. A. Janus
You can also search for this author in PubMed Google Scholar
- G. Jarlskog
You can also search for this author in PubMed Google Scholar
- A. E. Jaspan
You can also search for this author in PubMed Google Scholar
- N. Javadov
You can also search for this author in PubMed Google Scholar
- T. Javůrek
You can also search for this author in PubMed Google Scholar
- M. Javurkova
You can also search for this author in PubMed Google Scholar
- F. Jeanneau
You can also search for this author in PubMed Google Scholar
- L. Jeanty
You can also search for this author in PubMed Google Scholar
- J. Jejelava
You can also search for this author in PubMed Google Scholar
- P. Jenni
You can also search for this author in PubMed Google Scholar
- N. Jeong
You can also search for this author in PubMed Google Scholar
- S. Jézéquel
You can also search for this author in PubMed Google Scholar
- J. Jia
You can also search for this author in PubMed Google Scholar
- Z. Jia
You can also search for this author in PubMed Google Scholar
- H. Jiang
You can also search for this author in PubMed Google Scholar
- Y. Jiang
You can also search for this author in PubMed Google Scholar
- Z. Jiang
You can also search for this author in PubMed Google Scholar
- S. Jiggins
You can also search for this author in PubMed Google Scholar
- F. A. Jimenez Morales
You can also search for this author in PubMed Google Scholar
- J. Jimenez Pena
You can also search for this author in PubMed Google Scholar
- S. **
You can also search for this author in PubMed Google Scholar
- A. **aru
You can also search for this author in PubMed Google Scholar
- O. **nouchi
You can also search for this author in PubMed Google Scholar
- H. Jivan
You can also search for this author in PubMed Google Scholar
- P. Johansson
You can also search for this author in PubMed Google Scholar
- K. A. Johns
You can also search for this author in PubMed Google Scholar
- C. A. Johnson
You can also search for this author in PubMed Google Scholar
- E. Jones
You can also search for this author in PubMed Google Scholar
- R. W. L. Jones
You can also search for this author in PubMed Google Scholar
- S. D. Jones
You can also search for this author in PubMed Google Scholar
- T. J. Jones
You can also search for this author in PubMed Google Scholar
- J. Jovicevic
You can also search for this author in PubMed Google Scholar
- X. Ju
You can also search for this author in PubMed Google Scholar
- J. J. Junggeburth
You can also search for this author in PubMed Google Scholar
- A. Juste Rozas
You can also search for this author in PubMed Google Scholar
- A. Kaczmarska
You can also search for this author in PubMed Google Scholar
- M. Kado
You can also search for this author in PubMed Google Scholar
- H. Kagan
You can also search for this author in PubMed Google Scholar
- M. Kagan
You can also search for this author in PubMed Google Scholar
- A. Kahn
You can also search for this author in PubMed Google Scholar
- C. Kahra
You can also search for this author in PubMed Google Scholar
- T. Kaji
You can also search for this author in PubMed Google Scholar
- E. Kajomovitz
You can also search for this author in PubMed Google Scholar
- C. W. Kalderon
You can also search for this author in PubMed Google Scholar
- A. Kaluza
You can also search for this author in PubMed Google Scholar
- A. Kamenshchikov
You can also search for this author in PubMed Google Scholar
- M. Kaneda
You can also search for this author in PubMed Google Scholar
- N. J. Kang
You can also search for this author in PubMed Google Scholar
- S. Kang
You can also search for this author in PubMed Google Scholar
- Y. Kano
You can also search for this author in PubMed Google Scholar
- J. Kanzaki
You can also search for this author in PubMed Google Scholar
- L. S. Kaplan
You can also search for this author in PubMed Google Scholar
- D. Kar
You can also search for this author in PubMed Google Scholar
- K. Karava
You can also search for this author in PubMed Google Scholar
- M. J. Kareem
You can also search for this author in PubMed Google Scholar
- I. Karkanias
You can also search for this author in PubMed Google Scholar
- S. N. Karpov
You can also search for this author in PubMed Google Scholar
- Z. M. Karpova
You can also search for this author in PubMed Google Scholar
- V. Kartvelishvili
You can also search for this author in PubMed Google Scholar
- A. N. Karyukhin
You can also search for this author in PubMed Google Scholar
- E. Kasimi
You can also search for this author in PubMed Google Scholar
- A. Kastanas
You can also search for this author in PubMed Google Scholar
- C. Kato
You can also search for this author in PubMed Google Scholar
- J. Katzy
You can also search for this author in PubMed Google Scholar
- K. Kawade
You can also search for this author in PubMed Google Scholar
- K. Kawagoe
You can also search for this author in PubMed Google Scholar
- T. Kawaguchi
You can also search for this author in PubMed Google Scholar
- T. Kawamoto
You can also search for this author in PubMed Google Scholar
- G. Kawamura
You can also search for this author in PubMed Google Scholar
- E. F. Kay
You can also search for this author in PubMed Google Scholar
- F. I. Kaya
You can also search for this author in PubMed Google Scholar
- S. Kazakos
You can also search for this author in PubMed Google Scholar
- V. F. Kazanin
You can also search for this author in PubMed Google Scholar
- J. M. Keaveney
You can also search for this author in PubMed Google Scholar
- R. Keeler
You can also search for this author in PubMed Google Scholar
- J. S. Keller
You can also search for this author in PubMed Google Scholar
- E. Kellermann
You can also search for this author in PubMed Google Scholar
- D. Kelsey
You can also search for this author in PubMed Google Scholar
- J. J. Kempster
You can also search for this author in PubMed Google Scholar
- J. Kendrick
You can also search for this author in PubMed Google Scholar
- K. E. Kennedy
You can also search for this author in PubMed Google Scholar
- O. Kepka
You can also search for this author in PubMed Google Scholar
- S. Kersten
You can also search for this author in PubMed Google Scholar
- B. P. Kerševan
You can also search for this author in PubMed Google Scholar
- S. Ketabchi Haghighat
You can also search for this author in PubMed Google Scholar
- F. Khalil-Zada
You can also search for this author in PubMed Google Scholar
- M. Khandoga
You can also search for this author in PubMed Google Scholar
- A. Khanov
You can also search for this author in PubMed Google Scholar
- A. G. Kharlamov
You can also search for this author in PubMed Google Scholar
- T. Kharlamova
You can also search for this author in PubMed Google Scholar
- E. E. Khoda
You can also search for this author in PubMed Google Scholar
- T. J. Khoo
You can also search for this author in PubMed Google Scholar
- G. Khoriauli
You can also search for this author in PubMed Google Scholar
- E. Khramov
You can also search for this author in PubMed Google Scholar
- J. Khubua
You can also search for this author in PubMed Google Scholar
- S. Kido
You can also search for this author in PubMed Google Scholar
- M. Kiehn
You can also search for this author in PubMed Google Scholar
- E. Kim
You can also search for this author in PubMed Google Scholar
- Y. K. Kim
You can also search for this author in PubMed Google Scholar
- N. Kimura
You can also search for this author in PubMed Google Scholar
- A. Kirchhoff
You can also search for this author in PubMed Google Scholar
- D. Kirchmeier
You can also search for this author in PubMed Google Scholar
- J. Kirk
You can also search for this author in PubMed Google Scholar
- A. E. Kiryunin
You can also search for this author in PubMed Google Scholar
- T. Kishimoto
You can also search for this author in PubMed Google Scholar
- D. P. Kisliuk
You can also search for this author in PubMed Google Scholar
- V. Kitali
You can also search for this author in PubMed Google Scholar
- C. Kitsaki
You can also search for this author in PubMed Google Scholar
- O. Kivernyk
You can also search for this author in PubMed Google Scholar
- T. Klapdor-Kleingrothaus
You can also search for this author in PubMed Google Scholar
- M. Klassen
You can also search for this author in PubMed Google Scholar
- C. Klein
You can also search for this author in PubMed Google Scholar
- M. H. Klein
You can also search for this author in PubMed Google Scholar
- M. Klein
You can also search for this author in PubMed Google Scholar
- U. Klein
You can also search for this author in PubMed Google Scholar
- K. Kleinknecht
You can also search for this author in PubMed Google Scholar
- P. Klimek
You can also search for this author in PubMed Google Scholar
- A. Klimentov
You can also search for this author in PubMed Google Scholar
- F. Klimpel
You can also search for this author in PubMed Google Scholar
- T. Klingl
You can also search for this author in PubMed Google Scholar
- T. Klioutchnikova
You can also search for this author in PubMed Google Scholar
- F. F. Klitzner
You can also search for this author in PubMed Google Scholar
- P. Kluit
You can also search for this author in PubMed Google Scholar
- S. Kluth
You can also search for this author in PubMed Google Scholar
- E. Kneringer
You can also search for this author in PubMed Google Scholar
- E. B. F. G. Knoops
You can also search for this author in PubMed Google Scholar
- A. Knue
You can also search for this author in PubMed Google Scholar
- D. Kobayashi
You can also search for this author in PubMed Google Scholar
- M. Kobel
You can also search for this author in PubMed Google Scholar
- M. Kocian
You can also search for this author in PubMed Google Scholar
- T. Kodama
You can also search for this author in PubMed Google Scholar
- P. Kodys
You can also search for this author in PubMed Google Scholar
- D. M. Koeck
You can also search for this author in PubMed Google Scholar
- P. T. Koenig
You can also search for this author in PubMed Google Scholar
- T. Koffas
You can also search for this author in PubMed Google Scholar
- N. M. Köhler
You can also search for this author in PubMed Google Scholar
- M. Kolb
You can also search for this author in PubMed Google Scholar
- I. Koletsou
You can also search for this author in PubMed Google Scholar
- T. Komarek
You can also search for this author in PubMed Google Scholar
- T. Kondo
You can also search for this author in PubMed Google Scholar
- K. Köneke
You can also search for this author in PubMed Google Scholar
- A. X. Y. Kong
You can also search for this author in PubMed Google Scholar
- A. C. König
You can also search for this author in PubMed Google Scholar
- T. Kono
You can also search for this author in PubMed Google Scholar
- V. Konstantinides
You can also search for this author in PubMed Google Scholar
- N. Konstantinidis
You can also search for this author in PubMed Google Scholar
- B. Konya
You can also search for this author in PubMed Google Scholar
- R. Kopeliansky
You can also search for this author in PubMed Google Scholar
- S. Koperny
You can also search for this author in PubMed Google Scholar
- K. Korcyl
You can also search for this author in PubMed Google Scholar
- K. Kordas
You can also search for this author in PubMed Google Scholar
- G. Koren
You can also search for this author in PubMed Google Scholar
- A. Korn
You can also search for this author in PubMed Google Scholar
- I. Korolkov
You can also search for this author in PubMed Google Scholar
- E. V. Korolkova
You can also search for this author in PubMed Google Scholar
- N. Korotkova
You can also search for this author in PubMed Google Scholar
- O. Kortner
You can also search for this author in PubMed Google Scholar
- S. Kortner
You can also search for this author in PubMed Google Scholar
- V. V. Kostyukhin
You can also search for this author in PubMed Google Scholar
- A. Kotsokechagia
You can also search for this author in PubMed Google Scholar
- A. Kotwal
You can also search for this author in PubMed Google Scholar
- A. Koulouris
You can also search for this author in PubMed Google Scholar
- A. Kourkoumeli-Charalampidi
You can also search for this author in PubMed Google Scholar
- C. Kourkoumelis
You can also search for this author in PubMed Google Scholar
- E. Kourlitis
You can also search for this author in PubMed Google Scholar
- V. Kouskoura
You can also search for this author in PubMed Google Scholar
- R. Kowalewski
You can also search for this author in PubMed Google Scholar
- W. Kozanecki
You can also search for this author in PubMed Google Scholar
- A. S. Kozhin
You can also search for this author in PubMed Google Scholar
- V. A. Kramarenko
You can also search for this author in PubMed Google Scholar
- G. Kramberger
You can also search for this author in PubMed Google Scholar
- D. Krasnopevtsev
You can also search for this author in PubMed Google Scholar
- M. W. Krasny
You can also search for this author in PubMed Google Scholar
- A. Krasznahorkay
You can also search for this author in PubMed Google Scholar
- D. Krauss
You can also search for this author in PubMed Google Scholar
- J. A. Kremer
You can also search for this author in PubMed Google Scholar
- J. Kretzschmar
You can also search for this author in PubMed Google Scholar
- K. Kreul
You can also search for this author in PubMed Google Scholar
- P. Krieger
You can also search for this author in PubMed Google Scholar
- F. Krieter
You can also search for this author in PubMed Google Scholar
- S. Krishnamurthy
You can also search for this author in PubMed Google Scholar
- A. Krishnan
You can also search for this author in PubMed Google Scholar
- M. Krivos
You can also search for this author in PubMed Google Scholar
- K. Krizka
You can also search for this author in PubMed Google Scholar
- K. Kroeninger
You can also search for this author in PubMed Google Scholar
- H. Kroha
You can also search for this author in PubMed Google Scholar
- J. Kroll
You can also search for this author in PubMed Google Scholar
- J. Kroll
You can also search for this author in PubMed Google Scholar
- K. S. Krowpman
You can also search for this author in PubMed Google Scholar
- U. Kruchonak
You can also search for this author in PubMed Google Scholar
- H. Krüger
You can also search for this author in PubMed Google Scholar
- N. Krumnack
You can also search for this author in PubMed Google Scholar
- M. C. Kruse
You can also search for this author in PubMed Google Scholar
- J. A. Krzysiak
You can also search for this author in PubMed Google Scholar
- A. Kubota
You can also search for this author in PubMed Google Scholar
- O. Kuchinskaia
You can also search for this author in PubMed Google Scholar
- S. Kuday
You can also search for this author in PubMed Google Scholar
- D. Kuechler
You can also search for this author in PubMed Google Scholar
- J. T. Kuechler
You can also search for this author in PubMed Google Scholar
- S. Kuehn
You can also search for this author in PubMed Google Scholar
- T. Kuhl
You can also search for this author in PubMed Google Scholar
- V. Kukhtin
You can also search for this author in PubMed Google Scholar
- Y. Kulchitsky
You can also search for this author in PubMed Google Scholar
- S. Kuleshov
You can also search for this author in PubMed Google Scholar
- Y. P. Kulinich
You can also search for this author in PubMed Google Scholar
- M. Kuna
You can also search for this author in PubMed Google Scholar
- A. Kupco
You can also search for this author in PubMed Google Scholar
- T. Kupfer
You can also search for this author in PubMed Google Scholar
- O. Kuprash
You can also search for this author in PubMed Google Scholar
- H. Kurashige
You can also search for this author in PubMed Google Scholar
- L. L. Kurchaninov
You can also search for this author in PubMed Google Scholar
- Y. A. Kurochkin
You can also search for this author in PubMed Google Scholar
- A. Kurova
You can also search for this author in PubMed Google Scholar
- M. G. Kurth
You can also search for this author in PubMed Google Scholar
- E. S. Kuwertz
You can also search for this author in PubMed Google Scholar
- M. Kuze
You can also search for this author in PubMed Google Scholar
- A. K. Kvam
You can also search for this author in PubMed Google Scholar
- J. Kvita
You can also search for this author in PubMed Google Scholar
- T. Kwan
You can also search for this author in PubMed Google Scholar
- C. Lacasta
You can also search for this author in PubMed Google Scholar
- F. Lacava
You can also search for this author in PubMed Google Scholar
- D. P. J. Lack
You can also search for this author in PubMed Google Scholar
- H. Lacker
You can also search for this author in PubMed Google Scholar
- D. Lacour
You can also search for this author in PubMed Google Scholar
- E. Ladygin
You can also search for this author in PubMed Google Scholar
- R. Lafaye
You can also search for this author in PubMed Google Scholar
- B. Laforge
You can also search for this author in PubMed Google Scholar
- T. Lagouri
You can also search for this author in PubMed Google Scholar
- S. Lai
You can also search for this author in PubMed Google Scholar
- I. K. Lakomiec
You can also search for this author in PubMed Google Scholar
- J. E. Lambert
You can also search for this author in PubMed Google Scholar
- S. Lammers
You can also search for this author in PubMed Google Scholar
- W. Lampl
You can also search for this author in PubMed Google Scholar
- C. Lampoudis
You can also search for this author in PubMed Google Scholar
- E. Lançon
You can also search for this author in PubMed Google Scholar
- U. Landgraf
You can also search for this author in PubMed Google Scholar
- M. P. J. Landon
You can also search for this author in PubMed Google Scholar
- V. S. Lang
You can also search for this author in PubMed Google Scholar
- J. C. Lange
You can also search for this author in PubMed Google Scholar
- R. J. Langenberg
You can also search for this author in PubMed Google Scholar
- A. J. Lankford
You can also search for this author in PubMed Google Scholar
- F. Lanni
You can also search for this author in PubMed Google Scholar
- K. Lantzsch
You can also search for this author in PubMed Google Scholar
- A. Lanza
You can also search for this author in PubMed Google Scholar
- A. Lapertosa
You can also search for this author in PubMed Google Scholar
- J. F. Laporte
You can also search for this author in PubMed Google Scholar
- T. Lari
You can also search for this author in PubMed Google Scholar
- F. Lasagni Manghi
You can also search for this author in PubMed Google Scholar
- M. Lassnig
You can also search for this author in PubMed Google Scholar
- V. Latonova
You can also search for this author in PubMed Google Scholar
- T. S. Lau
You can also search for this author in PubMed Google Scholar
- A. Laudrain
You can also search for this author in PubMed Google Scholar
- A. Laurier
You can also search for this author in PubMed Google Scholar
- M. Lavorgna
You can also search for this author in PubMed Google Scholar
- S. D. Lawlor
You can also search for this author in PubMed Google Scholar
- M. Lazzaroni
You can also search for this author in PubMed Google Scholar
- B. Le
You can also search for this author in PubMed Google Scholar
- E. Le Guirriec
You can also search for this author in PubMed Google Scholar
- A. Lebedev
You can also search for this author in PubMed Google Scholar
- M. LeBlanc
You can also search for this author in PubMed Google Scholar
- T. LeCompte
You can also search for this author in PubMed Google Scholar
- F. Ledroit-Guillon
You can also search for this author in PubMed Google Scholar
- A. C. A. Lee
You can also search for this author in PubMed Google Scholar
- C. A. Lee
You can also search for this author in PubMed Google Scholar
- G. R. Lee
You can also search for this author in PubMed Google Scholar
- L. Lee
You can also search for this author in PubMed Google Scholar
- S. C. Lee
You can also search for this author in PubMed Google Scholar
- S. Lee
You can also search for this author in PubMed Google Scholar
- B. Lefebvre
You can also search for this author in PubMed Google Scholar
- H. P. Lefebvre
You can also search for this author in PubMed Google Scholar
- M. Lefebvre
You can also search for this author in PubMed Google Scholar
- C. Leggett
You can also search for this author in PubMed Google Scholar
- K. Lehmann
You can also search for this author in PubMed Google Scholar
- N. Lehmann
You can also search for this author in PubMed Google Scholar
- G. Lehmann Miotto
You can also search for this author in PubMed Google Scholar
- W. A. Leight
You can also search for this author in PubMed Google Scholar
- A. Leisos
You can also search for this author in PubMed Google Scholar
- M. A. L. Leite
You can also search for this author in PubMed Google Scholar
- C. E. Leitgeb
You can also search for this author in PubMed Google Scholar
- R. Leitner
You can also search for this author in PubMed Google Scholar
- K. J. C. Leney
You can also search for this author in PubMed Google Scholar
- T. Lenz
You can also search for this author in PubMed Google Scholar
- S. Leone
You can also search for this author in PubMed Google Scholar
- C. Leonidopoulos
You can also search for this author in PubMed Google Scholar
- A. Leopold
You can also search for this author in PubMed Google Scholar
- C. Leroy
You can also search for this author in PubMed Google Scholar
- R. Les
You can also search for this author in PubMed Google Scholar
- C. G. Lester
You can also search for this author in PubMed Google Scholar
- M. Levchenko
You can also search for this author in PubMed Google Scholar
- J. Levêque
You can also search for this author in PubMed Google Scholar
- D. Levin
You can also search for this author in PubMed Google Scholar
- L. J. Levinson
You can also search for this author in PubMed Google Scholar
- D. J. Lewis
You can also search for this author in PubMed Google Scholar
- B. Li
You can also search for this author in PubMed Google Scholar
- B. Li
You can also search for this author in PubMed Google Scholar
- C-Q. Li
You can also search for this author in PubMed Google Scholar
- F. Li
You can also search for this author in PubMed Google Scholar
- H. Li
You can also search for this author in PubMed Google Scholar
- H. Li
You can also search for this author in PubMed Google Scholar
- J. Li
You can also search for this author in PubMed Google Scholar
- K. Li
You can also search for this author in PubMed Google Scholar
- L. Li
You can also search for this author in PubMed Google Scholar
- M. Li
You can also search for this author in PubMed Google Scholar
- Q. Y. Li
You can also search for this author in PubMed Google Scholar
- S. Li
You can also search for this author in PubMed Google Scholar
- X. Li
You can also search for this author in PubMed Google Scholar
- Y. Li
You can also search for this author in PubMed Google Scholar
- Z. Li
You can also search for this author in PubMed Google Scholar
- Z. Li
You can also search for this author in PubMed Google Scholar
- Z. Li
You can also search for this author in PubMed Google Scholar
- Z. Li
You can also search for this author in PubMed Google Scholar
- Z. Liang
You can also search for this author in PubMed Google Scholar
- M. Liberatore
You can also search for this author in PubMed Google Scholar
- B. Liberti
You can also search for this author in PubMed Google Scholar
- K. Lie
You can also search for this author in PubMed Google Scholar
- S. Lim
You can also search for this author in PubMed Google Scholar
- C. Y. Lin
You can also search for this author in PubMed Google Scholar
- K. Lin
You can also search for this author in PubMed Google Scholar
- R. A. Linck
You can also search for this author in PubMed Google Scholar
- R. E. Lindley
You can also search for this author in PubMed Google Scholar
- J. H. Lindon
You can also search for this author in PubMed Google Scholar
- A. Linss
You can also search for this author in PubMed Google Scholar
- A. L. Lionti
You can also search for this author in PubMed Google Scholar
- E. Lipeles
You can also search for this author in PubMed Google Scholar
- A. Lipniacka
You can also search for this author in PubMed Google Scholar
- T. M. Liss
You can also search for this author in PubMed Google Scholar
- A. Lister
You can also search for this author in PubMed Google Scholar
- J. D. Little
You can also search for this author in PubMed Google Scholar
- B. Liu
You can also search for this author in PubMed Google Scholar
- B. X. Liu
You can also search for this author in PubMed Google Scholar
- H. B. Liu
You can also search for this author in PubMed Google Scholar
- J. B. Liu
You can also search for this author in PubMed Google Scholar
- J. K. K. Liu
You can also search for this author in PubMed Google Scholar
- K. Liu
You can also search for this author in PubMed Google Scholar
- M. Liu
You can also search for this author in PubMed Google Scholar
- M. Y. Liu
You can also search for this author in PubMed Google Scholar
- P. Liu
You can also search for this author in PubMed Google Scholar
- X. Liu
You can also search for this author in PubMed Google Scholar
- Y. Liu
You can also search for this author in PubMed Google Scholar
- Y. Liu
You can also search for this author in PubMed Google Scholar
- Y. L. Liu
You can also search for this author in PubMed Google Scholar
- Y. W. Liu
You can also search for this author in PubMed Google Scholar
- M. Livan
You can also search for this author in PubMed Google Scholar
- A. Lleres
You can also search for this author in PubMed Google Scholar
- J. Llorente Merino
You can also search for this author in PubMed Google Scholar
- S. L. Lloyd
You can also search for this author in PubMed Google Scholar
- C. Y. Lo
You can also search for this author in PubMed Google Scholar
- E. M. Lobodzinska
You can also search for this author in PubMed Google Scholar
- P. Loch
You can also search for this author in PubMed Google Scholar
- S. Loffredo
You can also search for this author in PubMed Google Scholar
- T. Lohse
You can also search for this author in PubMed Google Scholar
- K. Lohwasser
You can also search for this author in PubMed Google Scholar
- M. Lokajicek
You can also search for this author in PubMed Google Scholar
- J. D. Long
You can also search for this author in PubMed Google Scholar
- R. E. Long
You can also search for this author in PubMed Google Scholar
- I. Longarini
You can also search for this author in PubMed Google Scholar
- L. Longo
You can also search for this author in PubMed Google Scholar
- I. Lopez Paz
You can also search for this author in PubMed Google Scholar
- A. Lopez Solis
You can also search for this author in PubMed Google Scholar
- J. Lorenz
You can also search for this author in PubMed Google Scholar
- N. Lorenzo Martinez
You can also search for this author in PubMed Google Scholar
- A. M. Lory
You can also search for this author in PubMed Google Scholar
- A. Lösle
You can also search for this author in PubMed Google Scholar
- X. Lou
You can also search for this author in PubMed Google Scholar
- X. Lou
You can also search for this author in PubMed Google Scholar
- A. Lounis
You can also search for this author in PubMed Google Scholar
- J. Love
You can also search for this author in PubMed Google Scholar
- P. A. Love
You can also search for this author in PubMed Google Scholar
- J. J. Lozano Bahilo
You can also search for this author in PubMed Google Scholar
- M. Lu
You can also search for this author in PubMed Google Scholar
- Y. J. Lu
You can also search for this author in PubMed Google Scholar
- H. J. Lubatti
You can also search for this author in PubMed Google Scholar
- C. Luci
You can also search for this author in PubMed Google Scholar
- F. L. Lucio Alves
You can also search for this author in PubMed Google Scholar
- A. Lucotte
You can also search for this author in PubMed Google Scholar
- F. Luehring
You can also search for this author in PubMed Google Scholar
- I. Luise
You can also search for this author in PubMed Google Scholar
- L. Luminari
You can also search for this author in PubMed Google Scholar
- B. Lund-Jensen
You can also search for this author in PubMed Google Scholar
- N. A. Luongo
You can also search for this author in PubMed Google Scholar
- M. S. Lutz
You can also search for this author in PubMed Google Scholar
- D. Lynn
You can also search for this author in PubMed Google Scholar
- H. Lyons
You can also search for this author in PubMed Google Scholar
- R. Lysak
You can also search for this author in PubMed Google Scholar
- E. Lytken
You can also search for this author in PubMed Google Scholar
- F. Lyu
You can also search for this author in PubMed Google Scholar
- V. Lyubushkin
You can also search for this author in PubMed Google Scholar
- T. Lyubushkina
You can also search for this author in PubMed Google Scholar
- H. Ma
You can also search for this author in PubMed Google Scholar
- L. L. Ma
You can also search for this author in PubMed Google Scholar
- Y. Ma
You can also search for this author in PubMed Google Scholar
- D. M. Mac Donell
You can also search for this author in PubMed Google Scholar
- G. Maccarrone
You can also search for this author in PubMed Google Scholar
- C. M. Macdonald
You can also search for this author in PubMed Google Scholar
- J. C. MacDonald
You can also search for this author in PubMed Google Scholar
- J. Machado Miguens
You can also search for this author in PubMed Google Scholar
- R. Madar
You can also search for this author in PubMed Google Scholar
- W. F. Mader
You can also search for this author in PubMed Google Scholar
- M. Madugoda Ralalage Don
You can also search for this author in PubMed Google Scholar
- N. Madysa
You can also search for this author in PubMed Google Scholar
- J. Maeda
You can also search for this author in PubMed Google Scholar
- T. Maeno
You can also search for this author in PubMed Google Scholar
- M. Maerker
You can also search for this author in PubMed Google Scholar
- V. Magerl
You can also search for this author in PubMed Google Scholar
- N. Magini
You can also search for this author in PubMed Google Scholar
- J. Magro
You can also search for this author in PubMed Google Scholar
- D. J. Mahon
You can also search for this author in PubMed Google Scholar
- C. Maidantchik
You can also search for this author in PubMed Google Scholar
- A. Maio
You can also search for this author in PubMed Google Scholar
- K. Maj
You can also search for this author in PubMed Google Scholar
- O. Majersky
You can also search for this author in PubMed Google Scholar
- S. Majewski
You can also search for this author in PubMed Google Scholar
- Y. Makida
You can also search for this author in PubMed Google Scholar
- N. Makovec
You can also search for this author in PubMed Google Scholar
- B. Malaescu
You can also search for this author in PubMed Google Scholar
- Pa. Malecki
You can also search for this author in PubMed Google Scholar
- V. P. Maleev
You can also search for this author in PubMed Google Scholar
- F. Malek
You can also search for this author in PubMed Google Scholar
- D. Malito
You can also search for this author in PubMed Google Scholar
- U. Mallik
You can also search for this author in PubMed Google Scholar
- C. Malone
You can also search for this author in PubMed Google Scholar
- S. Maltezos
You can also search for this author in PubMed Google Scholar
- S. Malyukov
You can also search for this author in PubMed Google Scholar
- J. Mamuzic
You can also search for this author in PubMed Google Scholar
- G. Mancini
You can also search for this author in PubMed Google Scholar
- J. P. Mandalia
You can also search for this author in PubMed Google Scholar
- I. Mandić
You can also search for this author in PubMed Google Scholar
- L. Manhaes de Andrade Filho
You can also search for this author in PubMed Google Scholar
- I. M. Maniatis
You can also search for this author in PubMed Google Scholar
- J. Manjarres Ramos
You can also search for this author in PubMed Google Scholar
- K. H. Mankinen
You can also search for this author in PubMed Google Scholar
- A. Mann
You can also search for this author in PubMed Google Scholar
- A. Manousos
You can also search for this author in PubMed Google Scholar
- B. Mansoulie
You can also search for this author in PubMed Google Scholar
- I. Manthos
You can also search for this author in PubMed Google Scholar
- S. Manzoni
You can also search for this author in PubMed Google Scholar
- A. Marantis
You can also search for this author in PubMed Google Scholar
- G. Marceca
You can also search for this author in PubMed Google Scholar
- L. Marchese
You can also search for this author in PubMed Google Scholar
- G. Marchiori
You can also search for this author in PubMed Google Scholar
- M. Marcisovsky
You can also search for this author in PubMed Google Scholar
- L. Marcoccia
You can also search for this author in PubMed Google Scholar
- C. Marcon
You can also search for this author in PubMed Google Scholar
- M. Marjanovic
You can also search for this author in PubMed Google Scholar
- Z. Marshall
You can also search for this author in PubMed Google Scholar
- M. U. F. Martensson
You can also search for this author in PubMed Google Scholar
- S. Marti-Garcia
You can also search for this author in PubMed Google Scholar
- C. B. Martin
You can also search for this author in PubMed Google Scholar
- T. A. Martin
You can also search for this author in PubMed Google Scholar
- V. J. Martin
You can also search for this author in PubMed Google Scholar
- B. Martin dit Latour
You can also search for this author in PubMed Google Scholar
- L. Martinelli
You can also search for this author in PubMed Google Scholar
- M. Martinez
You can also search for this author in PubMed Google Scholar
- P. Martinez Agullo
You can also search for this author in PubMed Google Scholar
- V. I. Martinez Outschoorn
You can also search for this author in PubMed Google Scholar
- S. Martin-Haugh
You can also search for this author in PubMed Google Scholar
- V. S. Martoiu
You can also search for this author in PubMed Google Scholar
- A. C. Martyniuk
You can also search for this author in PubMed Google Scholar
- A. Marzin
You can also search for this author in PubMed Google Scholar
- S. R. Maschek
You can also search for this author in PubMed Google Scholar
- L. Masetti
You can also search for this author in PubMed Google Scholar
- T. Mashimo
You can also search for this author in PubMed Google Scholar
- R. Mashinistov
You can also search for this author in PubMed Google Scholar
- J. Masik
You can also search for this author in PubMed Google Scholar
- A. L. Maslennikov
You can also search for this author in PubMed Google Scholar
- L. Massa
You can also search for this author in PubMed Google Scholar
- P. Massarotti
You can also search for this author in PubMed Google Scholar
- P. Mastrandrea
You can also search for this author in PubMed Google Scholar
- A. Mastroberardino
You can also search for this author in PubMed Google Scholar
- T. Masubuchi
You can also search for this author in PubMed Google Scholar
- D. Matakias
You can also search for this author in PubMed Google Scholar
- A. Matic
You can also search for this author in PubMed Google Scholar
- N. Matsuzawa
You can also search for this author in PubMed Google Scholar
- P. Mättig
You can also search for this author in PubMed Google Scholar
- J. Maurer
You can also search for this author in PubMed Google Scholar
- B. Maček
You can also search for this author in PubMed Google Scholar
- D. A. Maximov
You can also search for this author in PubMed Google Scholar
- R. Mazini
You can also search for this author in PubMed Google Scholar
- I. Maznas
You can also search for this author in PubMed Google Scholar
- S. M. Mazza
You can also search for this author in PubMed Google Scholar
- J. P. Mc Gowan
You can also search for this author in PubMed Google Scholar
- S. P. Mc Kee
You can also search for this author in PubMed Google Scholar
- T. G. McCarthy
You can also search for this author in PubMed Google Scholar
- W. P. McCormack
You can also search for this author in PubMed Google Scholar
- E. F. McDonald
You can also search for this author in PubMed Google Scholar
- A. E. McDougall
You can also search for this author in PubMed Google Scholar
- J. A. Mcfayden
You can also search for this author in PubMed Google Scholar
- G. Mchedlidze
You can also search for this author in PubMed Google Scholar
- M. A. McKay
You can also search for this author in PubMed Google Scholar
- K. D. McLean
You can also search for this author in PubMed Google Scholar
- S. J. McMahon
You can also search for this author in PubMed Google Scholar
- P. C. McNamara
You can also search for this author in PubMed Google Scholar
- C. J. McNicol
You can also search for this author in PubMed Google Scholar
- R. A. McPherson
You can also search for this author in PubMed Google Scholar
- J. E. Mdhluli
You can also search for this author in PubMed Google Scholar
- Z. A. Meadows
You can also search for this author in PubMed Google Scholar
- S. Meehan
You can also search for this author in PubMed Google Scholar
- T. Megy
You can also search for this author in PubMed Google Scholar
- S. Mehlhase
You can also search for this author in PubMed Google Scholar
- A. Mehta
You can also search for this author in PubMed Google Scholar
- B. Meirose
You can also search for this author in PubMed Google Scholar
- D. Melini
You can also search for this author in PubMed Google Scholar
- B. R. Mellado Garcia
You can also search for this author in PubMed Google Scholar
- J. D. Mellenthin
You can also search for this author in PubMed Google Scholar
- M. Melo
You can also search for this author in PubMed Google Scholar
- F. Meloni
You can also search for this author in PubMed Google Scholar
- A. Melzer
You can also search for this author in PubMed Google Scholar
- E. D. Mendes Gouveia
You can also search for this author in PubMed Google Scholar
- A. M. Mendes Jacques Da Costa
You can also search for this author in PubMed Google Scholar
- H. Y. Meng
You can also search for this author in PubMed Google Scholar
- L. Meng
You can also search for this author in PubMed Google Scholar
- X. T. Meng
You can also search for this author in PubMed Google Scholar
- S. Menke
You can also search for this author in PubMed Google Scholar
- E. Meoni
You can also search for this author in PubMed Google Scholar
- S. Mergelmeyer
You can also search for this author in PubMed Google Scholar
- S. A. M. Merkt
You can also search for this author in PubMed Google Scholar
- C. Merlassino
You can also search for this author in PubMed Google Scholar
- P. Mermod
You can also search for this author in PubMed Google Scholar
- L. Merola
You can also search for this author in PubMed Google Scholar
- C. Meroni
You can also search for this author in PubMed Google Scholar
- G. Merz
You can also search for this author in PubMed Google Scholar
- O. Meshkov
You can also search for this author in PubMed Google Scholar
- J. K. R. Meshreki
You can also search for this author in PubMed Google Scholar
- J. Metcalfe
You can also search for this author in PubMed Google Scholar
- A. S. Mete
You can also search for this author in PubMed Google Scholar
- C. Meyer
You can also search for this author in PubMed Google Scholar
- J-P. Meyer
You can also search for this author in PubMed Google Scholar
- M. Michetti
You can also search for this author in PubMed Google Scholar
- R. P. Middleton
You can also search for this author in PubMed Google Scholar
- L. Mijović
You can also search for this author in PubMed Google Scholar
- G. Mikenberg
You can also search for this author in PubMed Google Scholar
- M. Mikestikova
You can also search for this author in PubMed Google Scholar
- M. Mikuž
You can also search for this author in PubMed Google Scholar
- H. Mildner
You can also search for this author in PubMed Google Scholar
- A. Milic
You can also search for this author in PubMed Google Scholar
- C. D. Milke
You can also search for this author in PubMed Google Scholar
- D. W. Miller
You can also search for this author in PubMed Google Scholar
- L. S. Miller
You can also search for this author in PubMed Google Scholar
- A. Milov
You can also search for this author in PubMed Google Scholar
- D. A. Milstead
You can also search for this author in PubMed Google Scholar
- A. A. Minaenko
You can also search for this author in PubMed Google Scholar
- I. A. Minashvili
You can also search for this author in PubMed Google Scholar
- L. Mince
You can also search for this author in PubMed Google Scholar
- A. I. Mincer
You can also search for this author in PubMed Google Scholar
- B. Mindur
You can also search for this author in PubMed Google Scholar
- M. Mineev
You can also search for this author in PubMed Google Scholar
- Y. Minegishi
You can also search for this author in PubMed Google Scholar
- Y. Mino
You can also search for this author in PubMed Google Scholar
- L. M. Mir
You can also search for this author in PubMed Google Scholar
- M. Mironova
You can also search for this author in PubMed Google Scholar
- T. Mitani
You can also search for this author in PubMed Google Scholar
- J. Mitrevski
You can also search for this author in PubMed Google Scholar
- V. A. Mitsou
You can also search for this author in PubMed Google Scholar
- M. Mittal
You can also search for this author in PubMed Google Scholar
- O. Miu
You can also search for this author in PubMed Google Scholar
- A. Miucci
You can also search for this author in PubMed Google Scholar
- P. S. Miyagawa
You can also search for this author in PubMed Google Scholar
- A. Mizukami
You can also search for this author in PubMed Google Scholar
- J. U. Mjörnmark
You can also search for this author in PubMed Google Scholar
- T. Mkrtchyan
You can also search for this author in PubMed Google Scholar
- M. Mlynarikova
You can also search for this author in PubMed Google Scholar
- T. Moa
You can also search for this author in PubMed Google Scholar
- S. Mobius
You can also search for this author in PubMed Google Scholar
- K. Mochizuki
You can also search for this author in PubMed Google Scholar
- P. Moder
You can also search for this author in PubMed Google Scholar
- P. Mogg
You can also search for this author in PubMed Google Scholar
- S. Mohapatra
You can also search for this author in PubMed Google Scholar
- R. Moles-Valls
You can also search for this author in PubMed Google Scholar
- K. Mönig
You can also search for this author in PubMed Google Scholar
- E. Monnier
You can also search for this author in PubMed Google Scholar
- A. Montalbano
You can also search for this author in PubMed Google Scholar
- J. Montejo Berlingen
You can also search for this author in PubMed Google Scholar
- M. Montella
You can also search for this author in PubMed Google Scholar
- F. Monticelli
You can also search for this author in PubMed Google Scholar
- S. Monzani
You can also search for this author in PubMed Google Scholar
- N. Morange
You can also search for this author in PubMed Google Scholar
- A. L. Moreira De Carvalho
You can also search for this author in PubMed Google Scholar
- D. Moreno
You can also search for this author in PubMed Google Scholar
- M. Moreno Llácer
You can also search for this author in PubMed Google Scholar
- C. Moreno Martinez
You can also search for this author in PubMed Google Scholar
- P. Morettini
You can also search for this author in PubMed Google Scholar
- M. Morgenstern
You can also search for this author in PubMed Google Scholar
- S. Morgenstern
You can also search for this author in PubMed Google Scholar
- D. Mori
You can also search for this author in PubMed Google Scholar
- M. Morii
You can also search for this author in PubMed Google Scholar
- M. Morinaga
You can also search for this author in PubMed Google Scholar
- V. Morisbak
You can also search for this author in PubMed Google Scholar
- A. K. Morley
You can also search for this author in PubMed Google Scholar
- G. Mornacchi
You can also search for this author in PubMed Google Scholar
- A. P. Morris
You can also search for this author in PubMed Google Scholar
- L. Morvaj
You can also search for this author in PubMed Google Scholar
- P. Moschovakos
You can also search for this author in PubMed Google Scholar
- B. Moser
You can also search for this author in PubMed Google Scholar
- M. Mosidze
You can also search for this author in PubMed Google Scholar
- T. Moskalets
You can also search for this author in PubMed Google Scholar
- P. Moskvitina
You can also search for this author in PubMed Google Scholar
- J. Moss
You can also search for this author in PubMed Google Scholar
- E. J. W. Moyse
You can also search for this author in PubMed Google Scholar
- S. Muanza
You can also search for this author in PubMed Google Scholar
- J. Mueller
You can also search for this author in PubMed Google Scholar
- R. S. P. Mueller
You can also search for this author in PubMed Google Scholar
- D. Muenstermann
You can also search for this author in PubMed Google Scholar
- G. A. Mullier
You can also search for this author in PubMed Google Scholar
- D. P. Mungo
You can also search for this author in PubMed Google Scholar
- J. L. Munoz Martinez
You can also search for this author in PubMed Google Scholar
- F. J. Munoz Sanchez
You can also search for this author in PubMed Google Scholar
- P. Murin
You can also search for this author in PubMed Google Scholar
- W. J. Murray
You can also search for this author in PubMed Google Scholar
- A. Murrone
You can also search for this author in PubMed Google Scholar
- J. M. Muse
You can also search for this author in PubMed Google Scholar
- M. Muškinja
You can also search for this author in PubMed Google Scholar
- C. Mwewa
You can also search for this author in PubMed Google Scholar
- A. G. Myagkov
You can also search for this author in PubMed Google Scholar
- A. A. Myers
You can also search for this author in PubMed Google Scholar
- G. Myers
You can also search for this author in PubMed Google Scholar
- J. Myers
You can also search for this author in PubMed Google Scholar
- M. Myska
You can also search for this author in PubMed Google Scholar
- B. P. Nachman
You can also search for this author in PubMed Google Scholar
- O. Nackenhorst
You can also search for this author in PubMed Google Scholar
- A.Nag Nag
You can also search for this author in PubMed Google Scholar
- K. Nagai
You can also search for this author in PubMed Google Scholar
- K. Nagano
You can also search for this author in PubMed Google Scholar
- Y. Nagasaka
You can also search for this author in PubMed Google Scholar
- J. L. Nagle
You can also search for this author in PubMed Google Scholar
- E. Nagy
You can also search for this author in PubMed Google Scholar
- A. M. Nairz
You can also search for this author in PubMed Google Scholar
- Y. Nakahama
You can also search for this author in PubMed Google Scholar
- K. Nakamura
You can also search for this author in PubMed Google Scholar
- T. Nakamura
You can also search for this author in PubMed Google Scholar
- H. Nanjo
You can also search for this author in PubMed Google Scholar
- F. Napolitano
You can also search for this author in PubMed Google Scholar
- R. F. Naranjo Garcia
You can also search for this author in PubMed Google Scholar
- R. Narayan
You can also search for this author in PubMed Google Scholar
- I. Naryshkin
You can also search for this author in PubMed Google Scholar
- M. Naseri
You can also search for this author in PubMed Google Scholar
- T. Naumann
You can also search for this author in PubMed Google Scholar
- G. Navarro
You can also search for this author in PubMed Google Scholar
- P. Y. Nechaeva
You can also search for this author in PubMed Google Scholar
- F. Nechansky
You can also search for this author in PubMed Google Scholar
- T. J. Neep
You can also search for this author in PubMed Google Scholar
- A. Negri
You can also search for this author in PubMed Google Scholar
- M. Negrini
You can also search for this author in PubMed Google Scholar
- C. Nellist
You can also search for this author in PubMed Google Scholar
- C. Nelson
You can also search for this author in PubMed Google Scholar
- M. E. Nelson
You can also search for this author in PubMed Google Scholar
- S. Nemecek
You can also search for this author in PubMed Google Scholar
- M. Nessi
You can also search for this author in PubMed Google Scholar
- M. S. Neubauer
You can also search for this author in PubMed Google Scholar
- F. Neuhaus
You can also search for this author in PubMed Google Scholar
- M. Neumann
You can also search for this author in PubMed Google Scholar
- R. Newhouse
You can also search for this author in PubMed Google Scholar
- P. R. Newman
You can also search for this author in PubMed Google Scholar
- C. W. Ng
You can also search for this author in PubMed Google Scholar
- Y. S. Ng
You can also search for this author in PubMed Google Scholar
- Y. W. Y. Ng
You can also search for this author in PubMed Google Scholar
- B. Ngair
You can also search for this author in PubMed Google Scholar
- H. D. N. Nguyen
You can also search for this author in PubMed Google Scholar
- T. Nguyen Manh
You can also search for this author in PubMed Google Scholar
- E. Nibigira
You can also search for this author in PubMed Google Scholar
- R. B. Nickerson
You can also search for this author in PubMed Google Scholar
- R. Nicolaidou
You can also search for this author in PubMed Google Scholar
- D. S. Nielsen
You can also search for this author in PubMed Google Scholar
- J. Nielsen
You can also search for this author in PubMed Google Scholar
- M. Niemeyer
You can also search for this author in PubMed Google Scholar
- N. Nikiforou
You can also search for this author in PubMed Google Scholar
- V. Nikolaenko
You can also search for this author in PubMed Google Scholar
- I. Nikolic-Audit
You can also search for this author in PubMed Google Scholar
- K. Nikolopoulos
You can also search for this author in PubMed Google Scholar
- P. Nilsson
You can also search for this author in PubMed Google Scholar
- H. R. Nindhito
You can also search for this author in PubMed Google Scholar
- A. Nisati
You can also search for this author in PubMed Google Scholar
- N. Nishu
You can also search for this author in PubMed Google Scholar
- R. Nisius
You can also search for this author in PubMed Google Scholar
- I. Nitsche
You can also search for this author in PubMed Google Scholar
- T. Nitta
You can also search for this author in PubMed Google Scholar
- T. Nobe
You can also search for this author in PubMed Google Scholar
- D. L. Noel
You can also search for this author in PubMed Google Scholar
- Y. Noguchi
You can also search for this author in PubMed Google Scholar
- I. Nomidis
You can also search for this author in PubMed Google Scholar
- M. A. Nomura
You can also search for this author in PubMed Google Scholar
- M. Nordberg
You can also search for this author in PubMed Google Scholar
- J. Novak
You can also search for this author in PubMed Google Scholar
- T. Novak
You can also search for this author in PubMed Google Scholar
- O. Novgorodova
You can also search for this author in PubMed Google Scholar
- R. Novotny
You can also search for this author in PubMed Google Scholar
- L. Nozka
You can also search for this author in PubMed Google Scholar
- K. Ntekas
You can also search for this author in PubMed Google Scholar
- E. Nurse
You can also search for this author in PubMed Google Scholar
- F. G. Oakham
You can also search for this author in PubMed Google Scholar
- J. Ocariz
You can also search for this author in PubMed Google Scholar
- A. Ochi
You can also search for this author in PubMed Google Scholar
- I. Ochoa
You can also search for this author in PubMed Google Scholar
- J. P. Ochoa-Ricoux
You can also search for this author in PubMed Google Scholar
- K. O’Connor
You can also search for this author in PubMed Google Scholar
- S. Oda
You can also search for this author in PubMed Google Scholar
- S. Odaka
You can also search for this author in PubMed Google Scholar
- S. Oerdek
You can also search for this author in PubMed Google Scholar
- A. Ogrodnik
You can also search for this author in PubMed Google Scholar
- A. Oh
You can also search for this author in PubMed Google Scholar
- C. C. Ohm
You can also search for this author in PubMed Google Scholar
- H. Oide
You can also search for this author in PubMed Google Scholar
- R. Oishi
You can also search for this author in PubMed Google Scholar
- M. L. Ojeda
You can also search for this author in PubMed Google Scholar
- H. Okawa
You can also search for this author in PubMed Google Scholar
- Y. Okazaki
You can also search for this author in PubMed Google Scholar
- M. W. O’Keefe
You can also search for this author in PubMed Google Scholar
- Y. Okumura
You can also search for this author in PubMed Google Scholar
- A. Olariu
You can also search for this author in PubMed Google Scholar
- L. F. Oleiro Seabra
You can also search for this author in PubMed Google Scholar
- S. A. Olivares Pino
You can also search for this author in PubMed Google Scholar
- D. Oliveira Damazio
You can also search for this author in PubMed Google Scholar
- J. L. Oliver
You can also search for this author in PubMed Google Scholar
- M. J. R. Olsson
You can also search for this author in PubMed Google Scholar
- A. Olszewski
You can also search for this author in PubMed Google Scholar
- J. Olszowska
You can also search for this author in PubMed Google Scholar
- Ö.O. Öncel
You can also search for this author in PubMed Google Scholar
- D. C. O’Neil
You can also search for this author in PubMed Google Scholar
- A. P. O’neill
You can also search for this author in PubMed Google Scholar
- A. Onofre
You can also search for this author in PubMed Google Scholar
- P. U. E. Onyisi
You can also search for this author in PubMed Google Scholar
- H. Oppen
You can also search for this author in PubMed Google Scholar
- R. G. Oreamuno Madriz
You can also search for this author in PubMed Google Scholar
- M. J. Oreglia
You can also search for this author in PubMed Google Scholar
- G. E. Orellana
You can also search for this author in PubMed Google Scholar
- D. Orestano
You can also search for this author in PubMed Google Scholar
- N. Orlando
You can also search for this author in PubMed Google Scholar
- R. S. Orr
You can also search for this author in PubMed Google Scholar
- V. O’Shea
You can also search for this author in PubMed Google Scholar
- R. Ospanov
You can also search for this author in PubMed Google Scholar
- G. Otero y Garzon
You can also search for this author in PubMed Google Scholar
- H. Otono
You can also search for this author in PubMed Google Scholar
- P. S. Ott
You can also search for this author in PubMed Google Scholar
- G. J. Ottino
You can also search for this author in PubMed Google Scholar
- M. Ouchrif
You can also search for this author in PubMed Google Scholar
- J. Ouellette
You can also search for this author in PubMed Google Scholar
- F. Ould-Saada
You can also search for this author in PubMed Google Scholar
- A. Ouraou
You can also search for this author in PubMed Google Scholar
- Q. Ouyang
You can also search for this author in PubMed Google Scholar
- M. Owen
You can also search for this author in PubMed Google Scholar
- R. E. Owen
You can also search for this author in PubMed Google Scholar
- V. E. Ozcan
You can also search for this author in PubMed Google Scholar
- N. Ozturk
You can also search for this author in PubMed Google Scholar
- J. Pacalt
You can also search for this author in PubMed Google Scholar
- H. A. Pacey
You can also search for this author in PubMed Google Scholar
- K. Pachal
You can also search for this author in PubMed Google Scholar
- A. Pacheco Pages
You can also search for this author in PubMed Google Scholar
- C. Padilla Aranda
You can also search for this author in PubMed Google Scholar
- S. Pagan Griso
You can also search for this author in PubMed Google Scholar
- G. Palacino
You can also search for this author in PubMed Google Scholar
- S. Palazzo
You can also search for this author in PubMed Google Scholar
- S. Palestini
You can also search for this author in PubMed Google Scholar
- M. Palka
You can also search for this author in PubMed Google Scholar
- P. Palni
You can also search for this author in PubMed Google Scholar
- C. E. Pandini
You can also search for this author in PubMed Google Scholar
- J. G. Panduro Vazquez
You can also search for this author in PubMed Google Scholar
- P. Pani
You can also search for this author in PubMed Google Scholar
- G. Panizzo
You can also search for this author in PubMed Google Scholar
- L. Paolozzi
You can also search for this author in PubMed Google Scholar
- C. Papadatos
You can also search for this author in PubMed Google Scholar
- K. Papageorgiou
You can also search for this author in PubMed Google Scholar
- S. Parajuli
You can also search for this author in PubMed Google Scholar
- A. Paramonov
You can also search for this author in PubMed Google Scholar
- C. Paraskevopoulos
You can also search for this author in PubMed Google Scholar
- D. Paredes Hernandez
You can also search for this author in PubMed Google Scholar
- S. R. Paredes Saenz
You can also search for this author in PubMed Google Scholar
- B. Parida
You can also search for this author in PubMed Google Scholar
- T. H. Park
You can also search for this author in PubMed Google Scholar
- A. J. Parker
You can also search for this author in PubMed Google Scholar
- M. A. Parker
You can also search for this author in PubMed Google Scholar
- F. Parodi
You can also search for this author in PubMed Google Scholar
- E. W. Parrish
You can also search for this author in PubMed Google Scholar
- J. A. Parsons
You can also search for this author in PubMed Google Scholar
- U. Parzefall
You can also search for this author in PubMed Google Scholar
- L. Pascual Dominguez
You can also search for this author in PubMed Google Scholar
- V. R. Pascuzzi
You can also search for this author in PubMed Google Scholar
- J. M. P. Pasner
You can also search for this author in PubMed Google Scholar
- F. Pasquali
You can also search for this author in PubMed Google Scholar
- E. Pasqualucci
You can also search for this author in PubMed Google Scholar
- S. Passaggio
You can also search for this author in PubMed Google Scholar
- F. Pastore
You can also search for this author in PubMed Google Scholar
- P. Pasuwan
You can also search for this author in PubMed Google Scholar
- S. Pataraia
You can also search for this author in PubMed Google Scholar
- J. R. Pater
You can also search for this author in PubMed Google Scholar
- A. Pathak
You can also search for this author in PubMed Google Scholar
- J. Patton
You can also search for this author in PubMed Google Scholar
- T. Pauly
You can also search for this author in PubMed Google Scholar
- J. Pearkes
You can also search for this author in PubMed Google Scholar
- M. Pedersen
You can also search for this author in PubMed Google Scholar
- L. Pedraza Diaz
You can also search for this author in PubMed Google Scholar
- R. Pedro
You can also search for this author in PubMed Google Scholar
- T. Peiffer
You can also search for this author in PubMed Google Scholar
- S. V. Peleganchuk
You can also search for this author in PubMed Google Scholar
- O. Penc
You can also search for this author in PubMed Google Scholar
- C. Peng
You can also search for this author in PubMed Google Scholar
- H. Peng
You can also search for this author in PubMed Google Scholar
- B. S. Peralva
You can also search for this author in PubMed Google Scholar
- M. M. Perego
You can also search for this author in PubMed Google Scholar
- A. P. Pereira Peixoto
You can also search for this author in PubMed Google Scholar
- L. Pereira Sanchez
You can also search for this author in PubMed Google Scholar
- D. V. Perepelitsa
You can also search for this author in PubMed Google Scholar
- E. Perez Codina
You can also search for this author in PubMed Google Scholar
- L. Perini
You can also search for this author in PubMed Google Scholar
- H. Pernegger
You can also search for this author in PubMed Google Scholar
- S. Perrella
You can also search for this author in PubMed Google Scholar
- A. Perrevoort
You can also search for this author in PubMed Google Scholar
- K. Peters
You can also search for this author in PubMed Google Scholar
- R. F. Y. Peters
You can also search for this author in PubMed Google Scholar
- B. A. Petersen
You can also search for this author in PubMed Google Scholar
- T. C. Petersen
You can also search for this author in PubMed Google Scholar
- E. Petit
You can also search for this author in PubMed Google Scholar
- V. Petousis
You can also search for this author in PubMed Google Scholar
- C. Petridou
You can also search for this author in PubMed Google Scholar
- F. Petrucci
You can also search for this author in PubMed Google Scholar
- M. Pettee
You can also search for this author in PubMed Google Scholar
- N. E. Pettersson
You can also search for this author in PubMed Google Scholar
- K. Petukhova
You can also search for this author in PubMed Google Scholar
- A. Peyaud
You can also search for this author in PubMed Google Scholar
- R. Pezoa
You can also search for this author in PubMed Google Scholar
- L. Pezzotti
You can also search for this author in PubMed Google Scholar
- T. Pham
You can also search for this author in PubMed Google Scholar
- P. W. Phillips
You can also search for this author in PubMed Google Scholar
- M. W. Phipps
You can also search for this author in PubMed Google Scholar
- G. Piacquadio
You can also search for this author in PubMed Google Scholar
- E. Pianori
You can also search for this author in PubMed Google Scholar
- A. Picazio
You can also search for this author in PubMed Google Scholar
- R. H. Pickles
You can also search for this author in PubMed Google Scholar
- R. Piegaia
You can also search for this author in PubMed Google Scholar
- D. Pietreanu
You can also search for this author in PubMed Google Scholar
- J. E. Pilcher
You can also search for this author in PubMed Google Scholar
- A. D. Pilkington
You can also search for this author in PubMed Google Scholar
- M. Pinamonti
You can also search for this author in PubMed Google Scholar
- J. L. Pinfold
You can also search for this author in PubMed Google Scholar
- C. Pitman Donaldson
You can also search for this author in PubMed Google Scholar
- M. Pitt
You can also search for this author in PubMed Google Scholar
- L. Pizzimento
You can also search for this author in PubMed Google Scholar
- A. Pizzini
You can also search for this author in PubMed Google Scholar
- M.-A. Pleier
You can also search for this author in PubMed Google Scholar
- V. Plesanovs
You can also search for this author in PubMed Google Scholar
- V. Pleskot
You can also search for this author in PubMed Google Scholar
- E. Plotnikova
You can also search for this author in PubMed Google Scholar
- P. Podberezko
You can also search for this author in PubMed Google Scholar
- R. Poettgen
You can also search for this author in PubMed Google Scholar
- R. Poggi
You can also search for this author in PubMed Google Scholar
- L. Poggioli
You can also search for this author in PubMed Google Scholar
- I. Pogrebnyak
You can also search for this author in PubMed Google Scholar
- D. Pohl
You can also search for this author in PubMed Google Scholar
- I. Pokharel
You can also search for this author in PubMed Google Scholar
- G. Polesello
You can also search for this author in PubMed Google Scholar
- A. Poley
You can also search for this author in PubMed Google Scholar
- A. Policicchio
You can also search for this author in PubMed Google Scholar
- R. Polifka
You can also search for this author in PubMed Google Scholar
- A. Polini
You can also search for this author in PubMed Google Scholar
- C. S. Pollard
You can also search for this author in PubMed Google Scholar
- V. Polychronakos
You can also search for this author in PubMed Google Scholar
- D. Ponomarenko
You can also search for this author in PubMed Google Scholar
- L. Pontecorvo
You can also search for this author in PubMed Google Scholar
- S. Popa
You can also search for this author in PubMed Google Scholar
- G. A. Popeneciu
You can also search for this author in PubMed Google Scholar
- L. Portales
You can also search for this author in PubMed Google Scholar
- D. M. Portillo Quintero
You can also search for this author in PubMed Google Scholar
- S. Pospisil
You can also search for this author in PubMed Google Scholar
- K. Potamianos
You can also search for this author in PubMed Google Scholar
- I. N. Potrap
You can also search for this author in PubMed Google Scholar
- C. J. Potter
You can also search for this author in PubMed Google Scholar
- H. Potti
You can also search for this author in PubMed Google Scholar
- T. Poulsen
You can also search for this author in PubMed Google Scholar
- J. Poveda
You can also search for this author in PubMed Google Scholar
- T. D. Powell
You can also search for this author in PubMed Google Scholar
- G. Pownall
You can also search for this author in PubMed Google Scholar
- M. E. Pozo Astigarraga
You can also search for this author in PubMed Google Scholar
- A. Prades Ibanez
You can also search for this author in PubMed Google Scholar
- P. Pralavorio
You can also search for this author in PubMed Google Scholar
- M. M. Prapa
You can also search for this author in PubMed Google Scholar
- S. Prell
You can also search for this author in PubMed Google Scholar
- D. Price
You can also search for this author in PubMed Google Scholar
- M. Primavera
You can also search for this author in PubMed Google Scholar
- M. L. Proffitt
You can also search for this author in PubMed Google Scholar
- N. Proklova
You can also search for this author in PubMed Google Scholar
- K. Prokofiev
You can also search for this author in PubMed Google Scholar
- F. Prokoshin
You can also search for this author in PubMed Google Scholar
- S. Protopopescu
You can also search for this author in PubMed Google Scholar
- J. Proudfoot
You can also search for this author in PubMed Google Scholar
- M. Przybycien
You can also search for this author in PubMed Google Scholar
- D. Pudzha
You can also search for this author in PubMed Google Scholar
- A. Puri
You can also search for this author in PubMed Google Scholar
- P. Puzo
You can also search for this author in PubMed Google Scholar
- D. Pyatiizbyantseva
You can also search for this author in PubMed Google Scholar
- J. Qian
You can also search for this author in PubMed Google Scholar
- Y. Qin
You can also search for this author in PubMed Google Scholar
- A. Quadt
You can also search for this author in PubMed Google Scholar
- M. Queitsch-Maitland
You can also search for this author in PubMed Google Scholar
- G. Rabanal Bolanos
You can also search for this author in PubMed Google Scholar
- M. Racko
You can also search for this author in PubMed Google Scholar
- F. Ragusa
You can also search for this author in PubMed Google Scholar
- G. Rahal
You can also search for this author in PubMed Google Scholar
- J. A. Raine
You can also search for this author in PubMed Google Scholar
- S. Rajagopalan
You can also search for this author in PubMed Google Scholar
- A. Ramirez Morales
You can also search for this author in PubMed Google Scholar
- K. Ran
You can also search for this author in PubMed Google Scholar
- D. F. Rassloff
You can also search for this author in PubMed Google Scholar
- D. M. Rauch
You can also search for this author in PubMed Google Scholar
- F. Rauscher
You can also search for this author in PubMed Google Scholar
- S. Rave
You can also search for this author in PubMed Google Scholar
- B. Ravina
You can also search for this author in PubMed Google Scholar
- I. Ravinovich
You can also search for this author in PubMed Google Scholar
- J. H. Rawling
You can also search for this author in PubMed Google Scholar
- M. Raymond
You can also search for this author in PubMed Google Scholar
- A. L. Read
You can also search for this author in PubMed Google Scholar
- N. P. Readioff
You can also search for this author in PubMed Google Scholar
- M. Reale
You can also search for this author in PubMed Google Scholar
- D. M. Rebuzzi
You can also search for this author in PubMed Google Scholar
- G. Redlinger
You can also search for this author in PubMed Google Scholar
- K. Reeves
You can also search for this author in PubMed Google Scholar
- D. Reikher
You can also search for this author in PubMed Google Scholar
- A. Reiss
You can also search for this author in PubMed Google Scholar
- A. Rej
You can also search for this author in PubMed Google Scholar
- C. Rembser
You can also search for this author in PubMed Google Scholar
- A. Renardi
You can also search for this author in PubMed Google Scholar
- M. Renda
You can also search for this author in PubMed Google Scholar
- M. B. Rendel
You can also search for this author in PubMed Google Scholar
- A. G. Rennie
You can also search for this author in PubMed Google Scholar
- S. Resconi
You can also search for this author in PubMed Google Scholar
- E. D. Resseguie
You can also search for this author in PubMed Google Scholar
- S. Rettie
You can also search for this author in PubMed Google Scholar
- B. Reynolds
You can also search for this author in PubMed Google Scholar
- E. Reynolds
You can also search for this author in PubMed Google Scholar
- O. L. Rezanova
You can also search for this author in PubMed Google Scholar
- P. Reznicek
You can also search for this author in PubMed Google Scholar
- E. Ricci
You can also search for this author in PubMed Google Scholar
- R. Richter
You can also search for this author in PubMed Google Scholar
- S. Richter
You can also search for this author in PubMed Google Scholar
- E. Richter-Was
You can also search for this author in PubMed Google Scholar
- M. Ridel
You can also search for this author in PubMed Google Scholar
- P. Rieck
You can also search for this author in PubMed Google Scholar
- O. Rifki
You can also search for this author in PubMed Google Scholar
- M. Rijssenbeek
You can also search for this author in PubMed Google Scholar
- A. Rimoldi
You can also search for this author in PubMed Google Scholar
- M. Rimoldi
You can also search for this author in PubMed Google Scholar
- L. Rinaldi
You can also search for this author in PubMed Google Scholar
- T. T. Rinn
You can also search for this author in PubMed Google Scholar
- G. Ripellino
You can also search for this author in PubMed Google Scholar
- I. Riu
You can also search for this author in PubMed Google Scholar
- P. Rivadeneira
You can also search for this author in PubMed Google Scholar
- J. C. Rivera Vergara
You can also search for this author in PubMed Google Scholar
- F. Rizatdinova
You can also search for this author in PubMed Google Scholar
- E. Rizvi
You can also search for this author in PubMed Google Scholar
- C. Rizzi
You can also search for this author in PubMed Google Scholar
- S. H. Robertson
You can also search for this author in PubMed Google Scholar
- M. Robin
You can also search for this author in PubMed Google Scholar
- D. Robinson
You can also search for this author in PubMed Google Scholar
- C. M. Robles Gajardo
You can also search for this author in PubMed Google Scholar
- M. Robles Manzano
You can also search for this author in PubMed Google Scholar
- A. Robson
You can also search for this author in PubMed Google Scholar
- A. Rocchi
You can also search for this author in PubMed Google Scholar
- C. Roda
You can also search for this author in PubMed Google Scholar
- S. Rodriguez Bosca
You can also search for this author in PubMed Google Scholar
- A. Rodriguez Rodriguez
You can also search for this author in PubMed Google Scholar
- A. M. Rodríguez Vera
You can also search for this author in PubMed Google Scholar
- S. Roe
You can also search for this author in PubMed Google Scholar
- J. Roggel
You can also search for this author in PubMed Google Scholar
- O. Røhne
You can also search for this author in PubMed Google Scholar
- R. Röhrig
You can also search for this author in PubMed Google Scholar
- R. A. Rojas
You can also search for this author in PubMed Google Scholar
- B. Roland
You can also search for this author in PubMed Google Scholar
- C. P. A. Roland
You can also search for this author in PubMed Google Scholar
- J. Roloff
You can also search for this author in PubMed Google Scholar
- A. Romaniouk
You can also search for this author in PubMed Google Scholar
- M. Romano
You can also search for this author in PubMed Google Scholar
- N. Rompotis
You can also search for this author in PubMed Google Scholar
- M. Ronzani
You can also search for this author in PubMed Google Scholar
- L. Roos
You can also search for this author in PubMed Google Scholar
- S. Rosati
You can also search for this author in PubMed Google Scholar
- G. Rosin
You can also search for this author in PubMed Google Scholar
- B. J. Rosser
You can also search for this author in PubMed Google Scholar
- E. Rossi
You can also search for this author in PubMed Google Scholar
- E. Rossi
You can also search for this author in PubMed Google Scholar
- E. Rossi
You can also search for this author in PubMed Google Scholar
- L. P. Rossi
You can also search for this author in PubMed Google Scholar
- L. Rossini
You can also search for this author in PubMed Google Scholar
- R. Rosten
You can also search for this author in PubMed Google Scholar
- M. Rotaru
You can also search for this author in PubMed Google Scholar
- B. Rottler
You can also search for this author in PubMed Google Scholar
- D. Rousseau
You can also search for this author in PubMed Google Scholar
- G. Rovelli
You can also search for this author in PubMed Google Scholar
- A. Roy
You can also search for this author in PubMed Google Scholar
- D. Roy
You can also search for this author in PubMed Google Scholar
- A. Rozanov
You can also search for this author in PubMed Google Scholar
- Y. Rozen
You can also search for this author in PubMed Google Scholar
- X. Ruan
You can also search for this author in PubMed Google Scholar
- T. A. Ruggeri
You can also search for this author in PubMed Google Scholar
- F. Rühr
You can also search for this author in PubMed Google Scholar
- A. Ruiz-Martinez
You can also search for this author in PubMed Google Scholar
- A. Rummler
You can also search for this author in PubMed Google Scholar
- Z. Rurikova
You can also search for this author in PubMed Google Scholar
- N. A. Rusakovich
You can also search for this author in PubMed Google Scholar
- H. L. Russell
You can also search for this author in PubMed Google Scholar
- L. Rustige
You can also search for this author in PubMed Google Scholar
- J. P. Rutherfoord
You can also search for this author in PubMed Google Scholar
- E. M. Rüttinger
You can also search for this author in PubMed Google Scholar
- M. Rybar
You can also search for this author in PubMed Google Scholar
- G. Rybkin
You can also search for this author in PubMed Google Scholar
- E. B. Rye
You can also search for this author in PubMed Google Scholar
- A. Ryzhov
You can also search for this author in PubMed Google Scholar
- J. A. Sabater Iglesias
You can also search for this author in PubMed Google Scholar
- P. Sabatini
You can also search for this author in PubMed Google Scholar
- L. Sabetta
You can also search for this author in PubMed Google Scholar
- S. Sacerdoti
You can also search for this author in PubMed Google Scholar
- H.F-W. Sadrozinski
You can also search for this author in PubMed Google Scholar
- R. Sadykov
You can also search for this author in PubMed Google Scholar
- F. Safai Tehrani
You can also search for this author in PubMed Google Scholar
- B. Safarzadeh Samani
You can also search for this author in PubMed Google Scholar
- M. Safdari
You can also search for this author in PubMed Google Scholar
- P. Saha
You can also search for this author in PubMed Google Scholar
- S. Saha
You can also search for this author in PubMed Google Scholar
- M. Sahinsoy
You can also search for this author in PubMed Google Scholar
- A. Sahu
You can also search for this author in PubMed Google Scholar
- M. Saimpert
You can also search for this author in PubMed Google Scholar
- M. Saito
You can also search for this author in PubMed Google Scholar
- T. Saito
You can also search for this author in PubMed Google Scholar
- H. Sakamoto
You can also search for this author in PubMed Google Scholar
- D. Salamani
You can also search for this author in PubMed Google Scholar
- G. Salamanna
You can also search for this author in PubMed Google Scholar
- A. Salnikov
You can also search for this author in PubMed Google Scholar
- J. Salt
You can also search for this author in PubMed Google Scholar
- A. Salvador Salas
You can also search for this author in PubMed Google Scholar
- D. Salvatore
You can also search for this author in PubMed Google Scholar
- F. Salvatore
You can also search for this author in PubMed Google Scholar
- A. Salvucci
You can also search for this author in PubMed Google Scholar
- A. Salzburger
You can also search for this author in PubMed Google Scholar
- J. Samarati
You can also search for this author in PubMed Google Scholar
- D. Sammel
You can also search for this author in PubMed Google Scholar
- D. Sampsonidis
You can also search for this author in PubMed Google Scholar
- D. Sampsonidou
You can also search for this author in PubMed Google Scholar
- J. Sánchez
You can also search for this author in PubMed Google Scholar
- A. Sanchez Pineda
You can also search for this author in PubMed Google Scholar
- H. Sandaker
You can also search for this author in PubMed Google Scholar
- C. O. Sander
You can also search for this author in PubMed Google Scholar
- I. G. Sanderswood
You can also search for this author in PubMed Google Scholar
- M. Sandhoff
You can also search for this author in PubMed Google Scholar
- C. Sandoval
You can also search for this author in PubMed Google Scholar
- D. P. C. Sankey
You can also search for this author in PubMed Google Scholar
- M. Sannino
You can also search for this author in PubMed Google Scholar
- Y. Sano
You can also search for this author in PubMed Google Scholar
- A. Sansoni
You can also search for this author in PubMed Google Scholar
- C. Santoni
You can also search for this author in PubMed Google Scholar
- H. Santos
You can also search for this author in PubMed Google Scholar
- S. N. Santpur
You can also search for this author in PubMed Google Scholar
- A. Santra
You can also search for this author in PubMed Google Scholar
- K. A. Saoucha
You can also search for this author in PubMed Google Scholar
- A. Sapronov
You can also search for this author in PubMed Google Scholar
- J. G. Saraiva
You can also search for this author in PubMed Google Scholar
- O. Sasaki
You can also search for this author in PubMed Google Scholar
- K. Sato
You can also search for this author in PubMed Google Scholar
- F. Sauerburger
You can also search for this author in PubMed Google Scholar
- E. Sauvan
You can also search for this author in PubMed Google Scholar
- P. Savard
You can also search for this author in PubMed Google Scholar
- R. Sawada
You can also search for this author in PubMed Google Scholar
- C. Sawyer
You can also search for this author in PubMed Google Scholar
- L. Sawyer
You can also search for this author in PubMed Google Scholar
- I. Sayago Galvan
You can also search for this author in PubMed Google Scholar
- C. Sbarra
You can also search for this author in PubMed Google Scholar
- A. Sbrizzi
You can also search for this author in PubMed Google Scholar
- T. Scanlon
You can also search for this author in PubMed Google Scholar
- J. Schaarschmidt
You can also search for this author in PubMed Google Scholar
- P. Schacht
You can also search for this author in PubMed Google Scholar
- D. Schaefer
You can also search for this author in PubMed Google Scholar
- L. Schaefer
You can also search for this author in PubMed Google Scholar
- U. Schäfer
You can also search for this author in PubMed Google Scholar
- A. C. Schaffer
You can also search for this author in PubMed Google Scholar
- D. Schaile
You can also search for this author in PubMed Google Scholar
- R. D. Schamberger
You can also search for this author in PubMed Google Scholar
- E. Schanet
You can also search for this author in PubMed Google Scholar
- C. Scharf
You can also search for this author in PubMed Google Scholar
- N. Scharmberg
You can also search for this author in PubMed Google Scholar
- V. A. Schegelsky
You can also search for this author in PubMed Google Scholar
- D. Scheirich
You can also search for this author in PubMed Google Scholar
- F. Schenck
You can also search for this author in PubMed Google Scholar
- M. Schernau
You can also search for this author in PubMed Google Scholar
- C. Schiavi
You can also search for this author in PubMed Google Scholar
- L. K. Schildgen
You can also search for this author in PubMed Google Scholar
- Z. M. Schillaci
You can also search for this author in PubMed Google Scholar
- E. J. Schioppa
You can also search for this author in PubMed Google Scholar
- M. Schioppa
You can also search for this author in PubMed Google Scholar
- K. E. Schleicher
You can also search for this author in PubMed Google Scholar
- S. Schlenker
You can also search for this author in PubMed Google Scholar
- K. R. Schmidt-Sommerfeld
You can also search for this author in PubMed Google Scholar
- K. Schmieden
You can also search for this author in PubMed Google Scholar
- C. Schmitt
You can also search for this author in PubMed Google Scholar
- S. Schmitt
You can also search for this author in PubMed Google Scholar
- L. Schoeffel
You can also search for this author in PubMed Google Scholar
- A. Schoening
You can also search for this author in PubMed Google Scholar
- P. G. Scholer
You can also search for this author in PubMed Google Scholar
- E. Schopf
You can also search for this author in PubMed Google Scholar
- M. Schott
You can also search for this author in PubMed Google Scholar
- J. F. P. Schouwenberg
You can also search for this author in PubMed Google Scholar
- J. Schovancova
You can also search for this author in PubMed Google Scholar
- S. Schramm
You can also search for this author in PubMed Google Scholar
- F. Schroeder
You can also search for this author in PubMed Google Scholar
- A. Schulte
You can also search for this author in PubMed Google Scholar
- H-C. Schultz-Coulon
You can also search for this author in PubMed Google Scholar
- M. Schumacher
You can also search for this author in PubMed Google Scholar
- B. A. Schumm
You can also search for this author in PubMed Google Scholar
- Ph. Schune
You can also search for this author in PubMed Google Scholar
- A. Schwartzman
You can also search for this author in PubMed Google Scholar
- T. A. Schwarz
You can also search for this author in PubMed Google Scholar
- Ph. Schwemling
You can also search for this author in PubMed Google Scholar
- R. Schwienhorst
You can also search for this author in PubMed Google Scholar
- A. Sciandra
You can also search for this author in PubMed Google Scholar
- G. Sciolla
You can also search for this author in PubMed Google Scholar
- F. Scuri
You can also search for this author in PubMed Google Scholar
- F. Scutti
You can also search for this author in PubMed Google Scholar
- L. M. Scyboz
You can also search for this author in PubMed Google Scholar
- C. D. Sebastiani
You can also search for this author in PubMed Google Scholar
- K. Sedlaczek
You can also search for this author in PubMed Google Scholar
- P. Seema
You can also search for this author in PubMed Google Scholar
- S. C. Seidel
You can also search for this author in PubMed Google Scholar
- A. Seiden
You can also search for this author in PubMed Google Scholar
- B. D. Seidlitz
You can also search for this author in PubMed Google Scholar
- T. Seiss
You can also search for this author in PubMed Google Scholar
- C. Seitz
You can also search for this author in PubMed Google Scholar
- J. M. Seixas
You can also search for this author in PubMed Google Scholar
- G. Sekhniaidze
You can also search for this author in PubMed Google Scholar
- S. J. Sekula
You can also search for this author in PubMed Google Scholar
- N. Semprini-Cesari
You can also search for this author in PubMed Google Scholar
- S. Sen
You can also search for this author in PubMed Google Scholar
- C. Serfon
You can also search for this author in PubMed Google Scholar
- L. Serin
You can also search for this author in PubMed Google Scholar
- L. Serkin
You can also search for this author in PubMed Google Scholar
- M. Sessa
You can also search for this author in PubMed Google Scholar
- H. Severini
You can also search for this author in PubMed Google Scholar
- S. Sevova
You can also search for this author in PubMed Google Scholar
- F. Sforza
You can also search for this author in PubMed Google Scholar
- A. Sfyrla
You can also search for this author in PubMed Google Scholar
- E. Shabalina
You can also search for this author in PubMed Google Scholar
- J. D. Shahinian
You can also search for this author in PubMed Google Scholar
- N. W. Shaikh
You can also search for this author in PubMed Google Scholar
- D. Shaked Renous
You can also search for this author in PubMed Google Scholar
- L. Y. Shan
You can also search for this author in PubMed Google Scholar
- M. Shapiro
You can also search for this author in PubMed Google Scholar
- A. Sharma
You can also search for this author in PubMed Google Scholar
- A. S. Sharma
You can also search for this author in PubMed Google Scholar
- P. B. Shatalov
You can also search for this author in PubMed Google Scholar
- K. Shaw
You can also search for this author in PubMed Google Scholar
- S. M. Shaw
You can also search for this author in PubMed Google Scholar
- M. Shehade
You can also search for this author in PubMed Google Scholar
- Y. Shen
You can also search for this author in PubMed Google Scholar
- A. D. Sherman
You can also search for this author in PubMed Google Scholar
- P. Sherwood
You can also search for this author in PubMed Google Scholar
- L. Shi
You can also search for this author in PubMed Google Scholar
- C. O. Shimmin
You can also search for this author in PubMed Google Scholar
- Y. Shimogama
You can also search for this author in PubMed Google Scholar
- M. Shimojima
You can also search for this author in PubMed Google Scholar
- J. D. Shinner
You can also search for this author in PubMed Google Scholar
- I. P. J. Shipsey
You can also search for this author in PubMed Google Scholar
- S. Shirabe
You can also search for this author in PubMed Google Scholar
- M. Shiyakova
You can also search for this author in PubMed Google Scholar
- J. Shlomi
You can also search for this author in PubMed Google Scholar
- A. Shmeleva
You can also search for this author in PubMed Google Scholar
- M. J. Shochet
You can also search for this author in PubMed Google Scholar
- J. Shojaii
You can also search for this author in PubMed Google Scholar
- D. R. Shope
You can also search for this author in PubMed Google Scholar
- S. Shrestha
You can also search for this author in PubMed Google Scholar
- E. M. Shrif
You can also search for this author in PubMed Google Scholar
- M. J. Shroff
You can also search for this author in PubMed Google Scholar
- E. Shulga
You can also search for this author in PubMed Google Scholar
- P. Sicho
You can also search for this author in PubMed Google Scholar
- A. M. Sickles
You can also search for this author in PubMed Google Scholar
- E. Sideras Haddad
You can also search for this author in PubMed Google Scholar
- O. Sidiropoulou
You can also search for this author in PubMed Google Scholar
- A. Sidoti
You can also search for this author in PubMed Google Scholar
- F. Siegert
You can also search for this author in PubMed Google Scholar
- Dj. Sijacki
You can also search for this author in PubMed Google Scholar
- M. Jr. Silva
You can also search for this author in PubMed Google Scholar
- M. V. Silva Oliveira
You can also search for this author in PubMed Google Scholar
- S. B. Silverstein
You can also search for this author in PubMed Google Scholar
- S. Simion
You can also search for this author in PubMed Google Scholar
- R. Simoniello
You can also search for this author in PubMed Google Scholar
- C. J. Simpson-allsop
You can also search for this author in PubMed Google Scholar
- S. Simsek
You can also search for this author in PubMed Google Scholar
- P. Sinervo
You can also search for this author in PubMed Google Scholar
- V. Sinetckii
You can also search for this author in PubMed Google Scholar
- S. Singh
You can also search for this author in PubMed Google Scholar
- S. Sinha
You can also search for this author in PubMed Google Scholar
- M. Sioli
You can also search for this author in PubMed Google Scholar
- I. Siral
You can also search for this author in PubMed Google Scholar
- S. Yu. Sivoklokov
You can also search for this author in PubMed Google Scholar
- J. Sjölin
You can also search for this author in PubMed Google Scholar
- A. Skaf
You can also search for this author in PubMed Google Scholar
- E. Skorda
You can also search for this author in PubMed Google Scholar
- P. Skubic
You can also search for this author in PubMed Google Scholar
- M. Slawinska
You can also search for this author in PubMed Google Scholar
- K. Sliwa
You can also search for this author in PubMed Google Scholar
- V. Smakhtin
You can also search for this author in PubMed Google Scholar
- B. H. Smart
You can also search for this author in PubMed Google Scholar
- J. Smiesko
You can also search for this author in PubMed Google Scholar
- N. Smirnov
You can also search for this author in PubMed Google Scholar
- S. Yu. Smirnov
You can also search for this author in PubMed Google Scholar
- Y. Smirnov
You can also search for this author in PubMed Google Scholar
- L. N. Smirnova
You can also search for this author in PubMed Google Scholar
- O. Smirnova
You can also search for this author in PubMed Google Scholar
- E. A. Smith
You can also search for this author in PubMed Google Scholar
- H. A. Smith
You can also search for this author in PubMed Google Scholar
- M. Smizanska
You can also search for this author in PubMed Google Scholar
- K. Smolek
You can also search for this author in PubMed Google Scholar
- A. Smykiewicz
You can also search for this author in PubMed Google Scholar
- A. A. Snesarev
You can also search for this author in PubMed Google Scholar
- H. L. Snoek
You can also search for this author in PubMed Google Scholar
- I. M. Snyder
You can also search for this author in PubMed Google Scholar
- S. Snyder
You can also search for this author in PubMed Google Scholar
- R. Sobie
You can also search for this author in PubMed Google Scholar
- A. Soffer
You can also search for this author in PubMed Google Scholar
- A. Søgaard
You can also search for this author in PubMed Google Scholar
- F. Sohns
You can also search for this author in PubMed Google Scholar
- C. A. Solans Sanchez
You can also search for this author in PubMed Google Scholar
- E. Yu. Soldatov
You can also search for this author in PubMed Google Scholar
- U. Soldevila
You can also search for this author in PubMed Google Scholar
- A. A. Solodkov
You can also search for this author in PubMed Google Scholar
- A. Soloshenko
You can also search for this author in PubMed Google Scholar
- O. V. Solovyanov
You can also search for this author in PubMed Google Scholar
- V. Solovyev
You can also search for this author in PubMed Google Scholar
- P. Sommer
You can also search for this author in PubMed Google Scholar
- H. Son
You can also search for this author in PubMed Google Scholar
- A. Sonay
You can also search for this author in PubMed Google Scholar
- W. Song
You can also search for this author in PubMed Google Scholar
- W. Y. Song
You can also search for this author in PubMed Google Scholar
- A. Sopczak
You can also search for this author in PubMed Google Scholar
- A. L. Sopio
You can also search for this author in PubMed Google Scholar
- F. Sopkova
You can also search for this author in PubMed Google Scholar
- S. Sottocornola
You can also search for this author in PubMed Google Scholar
- R. Soualah
You can also search for this author in PubMed Google Scholar
- A. M. Soukharev
You can also search for this author in PubMed Google Scholar
- D. South
You can also search for this author in PubMed Google Scholar
- S. Spagnolo
You can also search for this author in PubMed Google Scholar
- M. Spalla
You can also search for this author in PubMed Google Scholar
- M. Spangenberg
You can also search for this author in PubMed Google Scholar
- F. Spanò
You can also search for this author in PubMed Google Scholar
- D. Sperlich
You can also search for this author in PubMed Google Scholar
- T. M. Spieker
You can also search for this author in PubMed Google Scholar
- G. Spigo
You can also search for this author in PubMed Google Scholar
- M. Spina
You can also search for this author in PubMed Google Scholar
- D. P. Spiteri
You can also search for this author in PubMed Google Scholar
- M. Spousta
You can also search for this author in PubMed Google Scholar
- A. Stabile
You can also search for this author in PubMed Google Scholar
- B. L. Stamas
You can also search for this author in PubMed Google Scholar
- R. Stamen
You can also search for this author in PubMed Google Scholar
- M. Stamenkovic
You can also search for this author in PubMed Google Scholar
- A. Stampekis
You can also search for this author in PubMed Google Scholar
- E. Stanecka
You can also search for this author in PubMed Google Scholar
- B. Stanislaus
You can also search for this author in PubMed Google Scholar
- M. M. Stanitzki
You can also search for this author in PubMed Google Scholar
- M. Stankaityte
You can also search for this author in PubMed Google Scholar
- B. Stapf
You can also search for this author in PubMed Google Scholar
- E. A. Starchenko
You can also search for this author in PubMed Google Scholar
- G. H. Stark
You can also search for this author in PubMed Google Scholar
- J. Stark
You can also search for this author in PubMed Google Scholar
- P. Staroba
You can also search for this author in PubMed Google Scholar
- P. Starovoitov
You can also search for this author in PubMed Google Scholar
- S. Stärz
You can also search for this author in PubMed Google Scholar
- R. Staszewski
You can also search for this author in PubMed Google Scholar
- G. Stavropoulos
You can also search for this author in PubMed Google Scholar
- M. Stegler
You can also search for this author in PubMed Google Scholar
- P. Steinberg
You can also search for this author in PubMed Google Scholar
- A. L. Steinhebel
You can also search for this author in PubMed Google Scholar
- B. Stelzer
You can also search for this author in PubMed Google Scholar
- H. J. Stelzer
You can also search for this author in PubMed Google Scholar
- O. Stelzer-Chilton
You can also search for this author in PubMed Google Scholar
- H. Stenzel
You can also search for this author in PubMed Google Scholar
- T. J. Stevenson
You can also search for this author in PubMed Google Scholar
- G. A. Stewart
You can also search for this author in PubMed Google Scholar
- M. C. Stockton
You can also search for this author in PubMed Google Scholar
- G. Stoicea
You can also search for this author in PubMed Google Scholar
- M. Stolarski
You can also search for this author in PubMed Google Scholar
- S. Stonjek
You can also search for this author in PubMed Google Scholar
- A. Straessner
You can also search for this author in PubMed Google Scholar
- J. Strandberg
You can also search for this author in PubMed Google Scholar
- S. Strandberg
You can also search for this author in PubMed Google Scholar
- M. Strauss
You can also search for this author in PubMed Google Scholar
- T. Strebler
You can also search for this author in PubMed Google Scholar
- P. Strizenec
You can also search for this author in PubMed Google Scholar
- R. Ströhmer
You can also search for this author in PubMed Google Scholar
- D. M. Strom
You can also search for this author in PubMed Google Scholar
- R. Stroynowski
You can also search for this author in PubMed Google Scholar
- A. Strubig
You can also search for this author in PubMed Google Scholar
- S. A. Stucci
You can also search for this author in PubMed Google Scholar
- B. Stugu
You can also search for this author in PubMed Google Scholar
- J. Stupak
You can also search for this author in PubMed Google Scholar
- N. A. Styles
You can also search for this author in PubMed Google Scholar
- D. Su
You can also search for this author in PubMed Google Scholar
- W. Su
You can also search for this author in PubMed Google Scholar
- X. Su
You can also search for this author in PubMed Google Scholar
- N. B. Suarez
You can also search for this author in PubMed Google Scholar
- V. V. Sulin
You can also search for this author in PubMed Google Scholar
- M. J. Sullivan
You can also search for this author in PubMed Google Scholar
- D. M. S. Sultan
You can also search for this author in PubMed Google Scholar
- S. Sultansoy
You can also search for this author in PubMed Google Scholar
- T. Sumida
You can also search for this author in PubMed Google Scholar
- S. Sun
You can also search for this author in PubMed Google Scholar
- X. Sun
You can also search for this author in PubMed Google Scholar
- C. J. E. Suster
You can also search for this author in PubMed Google Scholar
- M. R. Sutton
You can also search for this author in PubMed Google Scholar
- S. Suzuki
You can also search for this author in PubMed Google Scholar
- M. Svatos
You can also search for this author in PubMed Google Scholar
- M. Swiatlowski
You can also search for this author in PubMed Google Scholar
- S. P. Swift
You can also search for this author in PubMed Google Scholar
- T. Swirski
You can also search for this author in PubMed Google Scholar
- A. Sydorenko
You can also search for this author in PubMed Google Scholar
- I. Sykora
You can also search for this author in PubMed Google Scholar
- M. Sykora
You can also search for this author in PubMed Google Scholar
- T. Sykora
You can also search for this author in PubMed Google Scholar
- D. Ta
You can also search for this author in PubMed Google Scholar
- K. Tackmann
You can also search for this author in PubMed Google Scholar
- J. Taenzer
You can also search for this author in PubMed Google Scholar
- A. Taffard
You can also search for this author in PubMed Google Scholar
- R. Tafirout
You can also search for this author in PubMed Google Scholar
- E. Tagiev
You can also search for this author in PubMed Google Scholar
- R. H. M. Taibah
You can also search for this author in PubMed Google Scholar
- R. Takashima
You can also search for this author in PubMed Google Scholar
- K. Takeda
You can also search for this author in PubMed Google Scholar
- T. Takeshita
You can also search for this author in PubMed Google Scholar
- E. P. Takeva
You can also search for this author in PubMed Google Scholar
- Y. Takubo
You can also search for this author in PubMed Google Scholar
- M. Talby
You can also search for this author in PubMed Google Scholar
- A. A. Talyshev
You can also search for this author in PubMed Google Scholar
- K. C. Tam
You can also search for this author in PubMed Google Scholar
- N. M. Tamir
You can also search for this author in PubMed Google Scholar
- J. Tanaka
You can also search for this author in PubMed Google Scholar
- R. Tanaka
You can also search for this author in PubMed Google Scholar
- S. Tapia Araya
You can also search for this author in PubMed Google Scholar
- S. Tapprogge
You can also search for this author in PubMed Google Scholar
- A. Tarek Abouelfadl Mohamed
You can also search for this author in PubMed Google Scholar
- S. Tarem
You can also search for this author in PubMed Google Scholar
- K. Tariq
You can also search for this author in PubMed Google Scholar
- G. Tarna
You can also search for this author in PubMed Google Scholar
- G. F. Tartarelli
You can also search for this author in PubMed Google Scholar
- P. Tas
You can also search for this author in PubMed Google Scholar
- M. Tasevsky
You can also search for this author in PubMed Google Scholar
- E. Tassi
You can also search for this author in PubMed Google Scholar
- G. Tateno
You can also search for this author in PubMed Google Scholar
- A. Tavares Delgado
You can also search for this author in PubMed Google Scholar
- Y. Tayalati
You can also search for this author in PubMed Google Scholar
- A. J. Taylor
You can also search for this author in PubMed Google Scholar
- G. N. Taylor
You can also search for this author in PubMed Google Scholar
- W. Taylor
You can also search for this author in PubMed Google Scholar
- H. Teagle
You can also search for this author in PubMed Google Scholar
- A. S. Tee
You can also search for this author in PubMed Google Scholar
- R. Teixeira De Lima
You can also search for this author in PubMed Google Scholar
- P. Teixeira-Dias
You can also search for this author in PubMed Google Scholar
- H. Ten Kate
You can also search for this author in PubMed Google Scholar
- J. J. Teoh
You can also search for this author in PubMed Google Scholar
- K. Terashi
You can also search for this author in PubMed Google Scholar
- J. Terron
You can also search for this author in PubMed Google Scholar
- S. Terzo
You can also search for this author in PubMed Google Scholar
- M. Testa
You can also search for this author in PubMed Google Scholar
- R. J. Teuscher
You can also search for this author in PubMed Google Scholar
- N. Themistokleous
You can also search for this author in PubMed Google Scholar
- T. Theveneaux-Pelzer
You can also search for this author in PubMed Google Scholar
- D. W. Thomas
You can also search for this author in PubMed Google Scholar
- J. P. Thomas
You can also search for this author in PubMed Google Scholar
- E. A. Thompson
You can also search for this author in PubMed Google Scholar
- P. D. Thompson
You can also search for this author in PubMed Google Scholar
- E. Thomson
You can also search for this author in PubMed Google Scholar
- E. J. Thorpe
You can also search for this author in PubMed Google Scholar
- V. O. Tikhomirov
You can also search for this author in PubMed Google Scholar
- Yu. A. Tikhonov
You can also search for this author in PubMed Google Scholar
- S. Timoshenko
You can also search for this author in PubMed Google Scholar
- P. Tipton
You can also search for this author in PubMed Google Scholar
- S. Tisserant
You can also search for this author in PubMed Google Scholar
- K. Todome
You can also search for this author in PubMed Google Scholar
- S. Todorova-Nova
You can also search for this author in PubMed Google Scholar
- S. Todt
You can also search for this author in PubMed Google Scholar
- J. Tojo
You can also search for this author in PubMed Google Scholar
- S. Tokár
You can also search for this author in PubMed Google Scholar
- K. Tokushuku
You can also search for this author in PubMed Google Scholar
- E. Tolley
You can also search for this author in PubMed Google Scholar
- R. Tombs
You can also search for this author in PubMed Google Scholar
- K. G. Tomiwa
You can also search for this author in PubMed Google Scholar
- M. Tomoto
You can also search for this author in PubMed Google Scholar
- L. Tompkins
You can also search for this author in PubMed Google Scholar
- P. Tornambe
You can also search for this author in PubMed Google Scholar
- E. Torrence
You can also search for this author in PubMed Google Scholar
- H. Torres
You can also search for this author in PubMed Google Scholar
- E. Torró Pastor
You can also search for this author in PubMed Google Scholar
- M. Toscani
You can also search for this author in PubMed Google Scholar
- C. Tosciri
You can also search for this author in PubMed Google Scholar
- J. Toth
You can also search for this author in PubMed Google Scholar
- D. R. Tovey
You can also search for this author in PubMed Google Scholar
- A. Traeet
You can also search for this author in PubMed Google Scholar
- C. J. Treado
You can also search for this author in PubMed Google Scholar
- T. Trefzger
You can also search for this author in PubMed Google Scholar
- F. Tresoldi
You can also search for this author in PubMed Google Scholar
- A. Tricoli
You can also search for this author in PubMed Google Scholar
- I. M. Trigger
You can also search for this author in PubMed Google Scholar
- S. Trincaz-Duvoid
You can also search for this author in PubMed Google Scholar
- D. A. Trischuk
You can also search for this author in PubMed Google Scholar
- W. Trischuk
You can also search for this author in PubMed Google Scholar
- B. Trocmé
You can also search for this author in PubMed Google Scholar
- A. Trofymov
You can also search for this author in PubMed Google Scholar
- C. Troncon
You can also search for this author in PubMed Google Scholar
- F. Trovato
You can also search for this author in PubMed Google Scholar
- L. Truong
You can also search for this author in PubMed Google Scholar
- M. Trzebinski
You can also search for this author in PubMed Google Scholar
- A. Trzupek
You can also search for this author in PubMed Google Scholar
- F. Tsai
You can also search for this author in PubMed Google Scholar
- P. V. Tsiareshka
You can also search for this author in PubMed Google Scholar
- A. Tsirigotis
You can also search for this author in PubMed Google Scholar
- V. Tsiskaridze
You can also search for this author in PubMed Google Scholar
- E. G. Tskhadadze
You can also search for this author in PubMed Google Scholar
- M. Tsopoulou
You can also search for this author in PubMed Google Scholar
- I. I. Tsukerman
You can also search for this author in PubMed Google Scholar
- V. Tsulaia
You can also search for this author in PubMed Google Scholar
- S. Tsuno
You can also search for this author in PubMed Google Scholar
- D. Tsybychev
You can also search for this author in PubMed Google Scholar
- Y. Tu
You can also search for this author in PubMed Google Scholar
- A. Tudorache
You can also search for this author in PubMed Google Scholar
- V. Tudorache
You can also search for this author in PubMed Google Scholar
- A. N. Tuna
You can also search for this author in PubMed Google Scholar
- S. Turchikhin
You can also search for this author in PubMed Google Scholar
- D. Turgeman
You can also search for this author in PubMed Google Scholar
- I. Turk Cakir
You can also search for this author in PubMed Google Scholar
- R. J. Turner
You can also search for this author in PubMed Google Scholar
- R. Turra
You can also search for this author in PubMed Google Scholar
- P. M. Tuts
You can also search for this author in PubMed Google Scholar
- S. Tzamarias
You can also search for this author in PubMed Google Scholar
- E. Tzovara
You can also search for this author in PubMed Google Scholar
- K. Uchida
You can also search for this author in PubMed Google Scholar
- F. Ukegawa
You can also search for this author in PubMed Google Scholar
- G. Unal
You can also search for this author in PubMed Google Scholar
- M. Unal
You can also search for this author in PubMed Google Scholar
- A. Undrus
You can also search for this author in PubMed Google Scholar
- G. Unel
You can also search for this author in PubMed Google Scholar
- F. C. Ungaro
You can also search for this author in PubMed Google Scholar
- Y. Unno
You can also search for this author in PubMed Google Scholar
- K. Uno
You can also search for this author in PubMed Google Scholar
- J. Urban
You can also search for this author in PubMed Google Scholar
- P. Urquijo
You can also search for this author in PubMed Google Scholar
- G. Usai
You can also search for this author in PubMed Google Scholar
- Z. Uysal
You can also search for this author in PubMed Google Scholar
- V. Vacek
You can also search for this author in PubMed Google Scholar
- B. Vachon
You can also search for this author in PubMed Google Scholar
- K. O. H. Vadla
You can also search for this author in PubMed Google Scholar
- T. Vafeiadis
You can also search for this author in PubMed Google Scholar
- A. Vaidya
You can also search for this author in PubMed Google Scholar
- C. Valderanis
You can also search for this author in PubMed Google Scholar
- E. Valdes Santurio
You can also search for this author in PubMed Google Scholar
- M. Valente
You can also search for this author in PubMed Google Scholar
- S. Valentinetti
You can also search for this author in PubMed Google Scholar
- A. Valero
You can also search for this author in PubMed Google Scholar
- L. Valéry
You can also search for this author in PubMed Google Scholar
- R. A. Vallance
You can also search for this author in PubMed Google Scholar
- A. Vallier
You can also search for this author in PubMed Google Scholar
- J. A. Valls Ferrer
You can also search for this author in PubMed Google Scholar
- T. R. Van Daalen
You can also search for this author in PubMed Google Scholar
- P. Van Gemmeren
You can also search for this author in PubMed Google Scholar
- S. Van Stroud
You can also search for this author in PubMed Google Scholar
- I. Van Vulpen
You can also search for this author in PubMed Google Scholar
- M. Vanadia
You can also search for this author in PubMed Google Scholar
- W. Vandelli
You can also search for this author in PubMed Google Scholar
- M. Vandenbroucke
You can also search for this author in PubMed Google Scholar
- E. R. Vandewall
You can also search for this author in PubMed Google Scholar
- D. Vannicola
You can also search for this author in PubMed Google Scholar
- R. Vari
You can also search for this author in PubMed Google Scholar
- E. W. Varnes
You can also search for this author in PubMed Google Scholar
- C. Varni
You can also search for this author in PubMed Google Scholar
- T. Varol
You can also search for this author in PubMed Google Scholar
- D. Varouchas
You can also search for this author in PubMed Google Scholar
- K. E. Varvell
You can also search for this author in PubMed Google Scholar
- M. E. Vasile
You can also search for this author in PubMed Google Scholar
- G. A. Vasquez
You can also search for this author in PubMed Google Scholar
- F. Vazeille
You can also search for this author in PubMed Google Scholar
- D. Vazquez Furelos
You can also search for this author in PubMed Google Scholar
- T. Vazquez Schroeder
You can also search for this author in PubMed Google Scholar
- J. Veatch
You can also search for this author in PubMed Google Scholar
- V. Vecchio
You can also search for this author in PubMed Google Scholar
- M. J. Veen
You can also search for this author in PubMed Google Scholar
- L. M. Veloce
You can also search for this author in PubMed Google Scholar
- F. Veloso
You can also search for this author in PubMed Google Scholar
- S. Veneziano
You can also search for this author in PubMed Google Scholar
- A. Ventura
You can also search for this author in PubMed Google Scholar
- A. Verbytskyi
You can also search for this author in PubMed Google Scholar
- V. Vercesi
You can also search for this author in PubMed Google Scholar
- M. Verducci
You can also search for this author in PubMed Google Scholar
- C. M. Vergel Infante
You can also search for this author in PubMed Google Scholar
- C. Vergis
You can also search for this author in PubMed Google Scholar
- W. Verkerke
You can also search for this author in PubMed Google Scholar
- A. T. Vermeulen
You can also search for this author in PubMed Google Scholar
- J. C. Vermeulen
You can also search for this author in PubMed Google Scholar
- C. Vernieri
You can also search for this author in PubMed Google Scholar
- P. J. Verschuuren
You can also search for this author in PubMed Google Scholar
- M. C. Vetterli
You can also search for this author in PubMed Google Scholar
- N. Viaux Maira
You can also search for this author in PubMed Google Scholar
- T. Vickey
You can also search for this author in PubMed Google Scholar
- O. E. Vickey Boeriu
You can also search for this author in PubMed Google Scholar
- G. H. A. Viehhauser
You can also search for this author in PubMed Google Scholar
- L. Vigani
You can also search for this author in PubMed Google Scholar
- M. Villa
You can also search for this author in PubMed Google Scholar
- M. Villaplana Perez
You can also search for this author in PubMed Google Scholar
- E. M. Villhauer
You can also search for this author in PubMed Google Scholar
- E. Vilucchi
You can also search for this author in PubMed Google Scholar
- M. G. Vincter
You can also search for this author in PubMed Google Scholar
- G. S. Virdee
You can also search for this author in PubMed Google Scholar
- A. Vishwakarma
You can also search for this author in PubMed Google Scholar
- C. Vittori
You can also search for this author in PubMed Google Scholar
- I. Vivarelli
You can also search for this author in PubMed Google Scholar
- M. Vogel
You can also search for this author in PubMed Google Scholar
- P. Vokac
You can also search for this author in PubMed Google Scholar
- J. Von Ahnen
You can also search for this author in PubMed Google Scholar
- S. E. von Buddenbrock
You can also search for this author in PubMed Google Scholar
- E. Von Toerne
You can also search for this author in PubMed Google Scholar
- V. Vorobel
You can also search for this author in PubMed Google Scholar
- K. Vorobev
You can also search for this author in PubMed Google Scholar
- M. Vos
You can also search for this author in PubMed Google Scholar
- J. H. Vossebeld
You can also search for this author in PubMed Google Scholar
- M. Vozak
You can also search for this author in PubMed Google Scholar
- N. Vranjes
You can also search for this author in PubMed Google Scholar
- M. Vranjes Milosavljevic
You can also search for this author in PubMed Google Scholar
- V. Vrba
You can also search for this author in PubMed Google Scholar
- M. Vreeswijk
You can also search for this author in PubMed Google Scholar
- N. K. Vu
You can also search for this author in PubMed Google Scholar
- R. Vuillermet
You can also search for this author in PubMed Google Scholar
- I. Vukotic
You can also search for this author in PubMed Google Scholar
- S. Wada
You can also search for this author in PubMed Google Scholar
- P. Wagner
You can also search for this author in PubMed Google Scholar
- W. Wagner
You can also search for this author in PubMed Google Scholar
- J. Wagner-Kuhr
You can also search for this author in PubMed Google Scholar
- S. Wahdan
You can also search for this author in PubMed Google Scholar
- H. Wahlberg
You can also search for this author in PubMed Google Scholar
- R. Wakasa
You can also search for this author in PubMed Google Scholar
- V. M. Walbrecht
You can also search for this author in PubMed Google Scholar
- J. Walder
You can also search for this author in PubMed Google Scholar
- R. Walker
You can also search for this author in PubMed Google Scholar
- S. D. Walker
You can also search for this author in PubMed Google Scholar
- W. Walkowiak
You can also search for this author in PubMed Google Scholar
- V. Wallangen
You can also search for this author in PubMed Google Scholar
- A. M. Wang
You can also search for this author in PubMed Google Scholar
- A. Z. Wang
You can also search for this author in PubMed Google Scholar
- C. Wang
You can also search for this author in PubMed Google Scholar
- C. Wang
You can also search for this author in PubMed Google Scholar
- H. Wang
You can also search for this author in PubMed Google Scholar
- H. Wang
You can also search for this author in PubMed Google Scholar
- J. Wang
You can also search for this author in PubMed Google Scholar
- P. Wang
You can also search for this author in PubMed Google Scholar
- Q. Wang
You can also search for this author in PubMed Google Scholar
- R.-J. Wang
You can also search for this author in PubMed Google Scholar
- R. Wang
You can also search for this author in PubMed Google Scholar
- R. Wang
You can also search for this author in PubMed Google Scholar
- S. M. Wang
You can also search for this author in PubMed Google Scholar
- W. T. Wang
You can also search for this author in PubMed Google Scholar
- W. Wang
You can also search for this author in PubMed Google Scholar
- W. X. Wang
You can also search for this author in PubMed Google Scholar
- Y. Wang
You can also search for this author in PubMed Google Scholar
- Z. Wang
You can also search for this author in PubMed Google Scholar
- C. Wanotayaroj
You can also search for this author in PubMed Google Scholar
- A. Warburton
You can also search for this author in PubMed Google Scholar
- C. P. Ward
You can also search for this author in PubMed Google Scholar
- R. J. Ward
You can also search for this author in PubMed Google Scholar
- N. Warrack
You can also search for this author in PubMed Google Scholar
- A. T. Watson
You can also search for this author in PubMed Google Scholar
- M. F. Watson
You can also search for this author in PubMed Google Scholar
- G. Watts
You can also search for this author in PubMed Google Scholar
- B. M. Waugh
You can also search for this author in PubMed Google Scholar
- A. F. Webb
You can also search for this author in PubMed Google Scholar
- C. Weber
You can also search for this author in PubMed Google Scholar
- M. S. Weber
You can also search for this author in PubMed Google Scholar
- S. A. Weber
You can also search for this author in PubMed Google Scholar
- S. M. Weber
You can also search for this author in PubMed Google Scholar
- Y. Wei
You can also search for this author in PubMed Google Scholar
- A. R. Weidberg
You can also search for this author in PubMed Google Scholar
- J. Weingarten
You can also search for this author in PubMed Google Scholar
- M. Weirich
You can also search for this author in PubMed Google Scholar
- C. Weiser
You can also search for this author in PubMed Google Scholar
- P. S. Wells
You can also search for this author in PubMed Google Scholar
- T. Wenaus
You can also search for this author in PubMed Google Scholar
- B. Wendland
You can also search for this author in PubMed Google Scholar
- T. Wengler
You can also search for this author in PubMed Google Scholar
- S. Wenig
You can also search for this author in PubMed Google Scholar
- N. Wermes
You can also search for this author in PubMed Google Scholar
- M. Wessels
You can also search for this author in PubMed Google Scholar
- T. D. Weston
You can also search for this author in PubMed Google Scholar
- K. Whalen
You can also search for this author in PubMed Google Scholar
- A. M. Wharton
You can also search for this author in PubMed Google Scholar
- A. S. White
You can also search for this author in PubMed Google Scholar
- A. White
You can also search for this author in PubMed Google Scholar
- M. J. White
You can also search for this author in PubMed Google Scholar
- D. Whiteson
You can also search for this author in PubMed Google Scholar
- B. W. Whitmore
You can also search for this author in PubMed Google Scholar
- W. Wiedenmann
You can also search for this author in PubMed Google Scholar
- C. Wiel
You can also search for this author in PubMed Google Scholar
- M. Wielers
You can also search for this author in PubMed Google Scholar
- N. Wieseotte
You can also search for this author in PubMed Google Scholar
- C. Wiglesworth
You can also search for this author in PubMed Google Scholar
- L. A. M. Wiik-Fuchs
You can also search for this author in PubMed Google Scholar
- H. G. Wilkens
You can also search for this author in PubMed Google Scholar
- L. J. Wilkins
You can also search for this author in PubMed Google Scholar
- D. M. Williams
You can also search for this author in PubMed Google Scholar
- H. H. Williams
You can also search for this author in PubMed Google Scholar
- S. Williams
You can also search for this author in PubMed Google Scholar
- S. Willocq
You can also search for this author in PubMed Google Scholar
- P. J. Windischhofer
You can also search for this author in PubMed Google Scholar
- I. Wingerter-Seez
You can also search for this author in PubMed Google Scholar
- E. Winkels
You can also search for this author in PubMed Google Scholar
- F. Winklmeier
You can also search for this author in PubMed Google Scholar
- B. T. Winter
You can also search for this author in PubMed Google Scholar
- M. Wittgen
You can also search for this author in PubMed Google Scholar
- M. Wobisch
You can also search for this author in PubMed Google Scholar
- A. Wolf
You can also search for this author in PubMed Google Scholar
- R. Wölker
You can also search for this author in PubMed Google Scholar
- J. Wollrath
You can also search for this author in PubMed Google Scholar
- M. W. Wolter
You can also search for this author in PubMed Google Scholar
- H. Wolters
You can also search for this author in PubMed Google Scholar
- V. W. S. Wong
You can also search for this author in PubMed Google Scholar
- A. F. Wongel
You can also search for this author in PubMed Google Scholar
- N. L. Woods
You can also search for this author in PubMed Google Scholar
- S. D. Worm
You can also search for this author in PubMed Google Scholar
- B. K. Wosiek
You can also search for this author in PubMed Google Scholar
- K. W. Woźniak
You can also search for this author in PubMed Google Scholar
- K. Wraight
You can also search for this author in PubMed Google Scholar
- S. L. Wu
You can also search for this author in PubMed Google Scholar
- X. Wu
You can also search for this author in PubMed Google Scholar
- Y. Wu
You can also search for this author in PubMed Google Scholar
- J. Wuerzinger
You can also search for this author in PubMed Google Scholar
- T. R. Wyatt
You can also search for this author in PubMed Google Scholar
- B. M. Wynne
You can also search for this author in PubMed Google Scholar
- S. Xella
You can also search for this author in PubMed Google Scholar
- L. **a
You can also search for this author in PubMed Google Scholar
- J. **ang
You can also search for this author in PubMed Google Scholar
- X. **ao
You can also search for this author in PubMed Google Scholar
- X. **e
You can also search for this author in PubMed Google Scholar
- I. **otidis
You can also search for this author in PubMed Google Scholar
- D. Xu
You can also search for this author in PubMed Google Scholar
- H. Xu
You can also search for this author in PubMed Google Scholar
- H. Xu
You can also search for this author in PubMed Google Scholar
- L. Xu
You can also search for this author in PubMed Google Scholar
- R. Xu
You can also search for this author in PubMed Google Scholar
- T. Xu
You can also search for this author in PubMed Google Scholar
- W. Xu
You can also search for this author in PubMed Google Scholar
- Y. Xu
You can also search for this author in PubMed Google Scholar
- Z. Xu
You can also search for this author in PubMed Google Scholar
- Z. Xu
You can also search for this author in PubMed Google Scholar
- B. Yabsley
You can also search for this author in PubMed Google Scholar
- S. Yacoob
You can also search for this author in PubMed Google Scholar
- D. P. Yallup
You can also search for this author in PubMed Google Scholar
- N. Yamaguchi
You can also search for this author in PubMed Google Scholar
- Y. Yamaguchi
You can also search for this author in PubMed Google Scholar
- A. Yamamoto
You can also search for this author in PubMed Google Scholar
- M. Yamatani
You can also search for this author in PubMed Google Scholar
- T. Yamazaki
You can also search for this author in PubMed Google Scholar
- Y. Yamazaki
You can also search for this author in PubMed Google Scholar
- J. Yan
You can also search for this author in PubMed Google Scholar
- Z. Yan
You can also search for this author in PubMed Google Scholar
- H. J. Yang
You can also search for this author in PubMed Google Scholar
- H. T. Yang
You can also search for this author in PubMed Google Scholar
- S. Yang
You can also search for this author in PubMed Google Scholar
- T. Yang
You can also search for this author in PubMed Google Scholar
- X. Yang
You can also search for this author in PubMed Google Scholar
- X. Yang
You can also search for this author in PubMed Google Scholar
- Y. Yang
You can also search for this author in PubMed Google Scholar
- Z. Yang
You can also search for this author in PubMed Google Scholar
- W-M. Yao
You can also search for this author in PubMed Google Scholar
- Y. C. Yap
You can also search for this author in PubMed Google Scholar
- H. Ye
You can also search for this author in PubMed Google Scholar
- J. Ye
You can also search for this author in PubMed Google Scholar
- S. Ye
You can also search for this author in PubMed Google Scholar
- I. Yeletskikh
You can also search for this author in PubMed Google Scholar
- M. R. Yexley
You can also search for this author in PubMed Google Scholar
- E. Yigitbasi
You can also search for this author in PubMed Google Scholar
- P. Yin
You can also search for this author in PubMed Google Scholar
- K. Yorita
You can also search for this author in PubMed Google Scholar
- K. Yoshihara
You can also search for this author in PubMed Google Scholar
- C. J. S. Young
You can also search for this author in PubMed Google Scholar
- C. Young
You can also search for this author in PubMed Google Scholar
- J. Yu
You can also search for this author in PubMed Google Scholar
- R. Yuan
You can also search for this author in PubMed Google Scholar
- X. Yue
You can also search for this author in PubMed Google Scholar
- M. Zaazoua
You can also search for this author in PubMed Google Scholar
- B. Zabinski
You can also search for this author in PubMed Google Scholar
- G. Zacharis
You can also search for this author in PubMed Google Scholar
- E. Zaffaroni
You can also search for this author in PubMed Google Scholar
- J. Zahreddine
You can also search for this author in PubMed Google Scholar
- A. M. Zaitsev
You can also search for this author in PubMed Google Scholar
- T. Zakareishvili
You can also search for this author in PubMed Google Scholar
- N. Zakharchuk
You can also search for this author in PubMed Google Scholar
- S. Zambito
You can also search for this author in PubMed Google Scholar
- D. Zanzi
You can also search for this author in PubMed Google Scholar
- S. V. Zeißner
You can also search for this author in PubMed Google Scholar
- C. Zeitnitz
You can also search for this author in PubMed Google Scholar
- G. Zemaityte
You can also search for this author in PubMed Google Scholar
- J. C. Zeng
You can also search for this author in PubMed Google Scholar
- O. Zenin
You can also search for this author in PubMed Google Scholar
- T. Ženiš
You can also search for this author in PubMed Google Scholar
- D. Zerwas
You can also search for this author in PubMed Google Scholar
- M. Zgubič
You can also search for this author in PubMed Google Scholar
- B. Zhang
You can also search for this author in PubMed Google Scholar
- D. F. Zhang
You can also search for this author in PubMed Google Scholar
- G. Zhang
You can also search for this author in PubMed Google Scholar
- J. Zhang
You can also search for this author in PubMed Google Scholar
- K. Zhang
You can also search for this author in PubMed Google Scholar
- L. Zhang
You can also search for this author in PubMed Google Scholar
- L. Zhang
You can also search for this author in PubMed Google Scholar
- M. Zhang
You can also search for this author in PubMed Google Scholar
- R. Zhang
You can also search for this author in PubMed Google Scholar
- S. Zhang
You can also search for this author in PubMed Google Scholar
- X. Zhang
You can also search for this author in PubMed Google Scholar
- X. Zhang
You can also search for this author in PubMed Google Scholar
- Y. Zhang
You can also search for this author in PubMed Google Scholar
- Z. Zhang
You can also search for this author in PubMed Google Scholar
- Z. Zhang
You can also search for this author in PubMed Google Scholar
- P. Zhao
You can also search for this author in PubMed Google Scholar
- Y. Zhao
You can also search for this author in PubMed Google Scholar
- Z. Zhao
You can also search for this author in PubMed Google Scholar
- A. Zhemchugov
You can also search for this author in PubMed Google Scholar
- Z. Zheng
You can also search for this author in PubMed Google Scholar
- D. Zhong
You can also search for this author in PubMed Google Scholar
- B. Zhou
You can also search for this author in PubMed Google Scholar
- C. Zhou
You can also search for this author in PubMed Google Scholar
- H. Zhou
You can also search for this author in PubMed Google Scholar
- M. Zhou
You can also search for this author in PubMed Google Scholar
- N. Zhou
You can also search for this author in PubMed Google Scholar
- Y. Zhou
You can also search for this author in PubMed Google Scholar
- C. G. Zhu
You can also search for this author in PubMed Google Scholar
- C. Zhu
You can also search for this author in PubMed Google Scholar
- H. L. Zhu
You can also search for this author in PubMed Google Scholar
- H. Zhu
You can also search for this author in PubMed Google Scholar
- J. Zhu
You can also search for this author in PubMed Google Scholar
- Y. Zhu
You can also search for this author in PubMed Google Scholar
- X. Zhuang
You can also search for this author in PubMed Google Scholar
- K. Zhukov
You can also search for this author in PubMed Google Scholar
- V. Zhulanov
You can also search for this author in PubMed Google Scholar
- D. Zieminska
You can also search for this author in PubMed Google Scholar
- N. I. Zimine
You can also search for this author in PubMed Google Scholar
- S. Zimmermann
You can also search for this author in PubMed Google Scholar
- Z. Zinonos
You can also search for this author in PubMed Google Scholar
- M. Ziolkowski
You can also search for this author in PubMed Google Scholar
- L. Živković
You can also search for this author in PubMed Google Scholar
- G. Zobernig
You can also search for this author in PubMed Google Scholar
- A. Zoccoli
You can also search for this author in PubMed Google Scholar
- K. Zoch
You can also search for this author in PubMed Google Scholar
- T. G. Zorbas
You can also search for this author in PubMed Google Scholar
- R. Zou
You can also search for this author in PubMed Google Scholar
- L. Zwalinski
You can also search for this author in PubMed Google Scholar
Consortia
ATLAS Collaboration
Appendix
Appendix
Rights and permissions
Open Access This article is licensed under a Creative Commons Attribution 4.0 International License, which permits use, sharing, adaptation, distribution and reproduction in any medium or format, as long as you give appropriate credit to the original author(s) and the source, provide a link to the Creative Commons licence, and indicate if changes were made. The images or other third party material in this article are included in the article’s Creative Commons licence, unless indicated otherwise in a credit line to the material. If material is not included in the article’s Creative Commons licence and your intended use is not permitted by statutory regulation or exceeds the permitted use, you will need to obtain permission directly from the copyright holder. To view a copy of this licence, visit http://creativecommons.org/licenses/by/4.0/.
Funded by SCOAP3
About this article
Cite this article
Aad, G., Abbott, B., Abbott, D.C. et al. Search for pair production of scalar leptoquarks decaying into first- or second-generation leptons and top quarks in proton–proton collisions at \(\sqrt{s}\) = 13 TeV with the ATLAS detector. Eur. Phys. J. C 81, 313 (2021). https://doi.org/10.1140/epjc/s10052-021-09009-8
Received:
Accepted:
Published:
DOI: https://doi.org/10.1140/epjc/s10052-021-09009-8
Share this article
Anyone you share the following link with will be able to read this content:
Sorry, a shareable link is not currently available for this article.
Provided by the Springer Nature SharedIt content-sharing initiative