Abstract
Background
Seed aging, a natural and inevitable process occurring during storage. Oats, an annual herb belonging to the Gramineae family and pooideae. In addition to being a healthy food, oats serve as ecological pastures, combating soil salinization and desertification. They also play a role in promoting grassland agriculture and supplementing winter livestock feed. However, the high lipid and fat derivatives contents of oat seeds make them susceptible to deterioration, as fat derivatives are prone to rancidity, affecting oat seed production, storage, development, and germplasm resource utilization. Comparative studies on the effects of aging on physiology and cytological structure in covered and naked oat seeds are limited. Thus, our study aimed to determine the mechanism underlying seed deterioration in artificially aged ‘LongYan No. 3’ (A. sativa) and ‘BaiYan No. 2’ (A. nuda) seeds, providing a basis for the physiological evaluation of oat seed aging and serving as a reference for scientifically safe storage and efficient utilization of oats.
Results
In both oat varieties, superoxide dismutase and catalase activities in seeds showed increasing and decreasing trends, respectively. Variance analysis revealed significant differences and interaction in all measured indicators of oat seeds between the two varieties at different aging times. ‘LongYan No. 3’ seeds, aged for 24–96 h, exhibited a germination rate of < 30%, Conductivity, malondialdehyde, soluble sugar, and soluble protein levels increased more significantly than the ‘BaiYan No. 2’. With prolonged aging leading to cell membrane degradation, reactive oxygen species accumulation, disrupted antioxidant enzyme system, evident embryo cell swelling, and disordered cell arrangement, blocking the nutrient supply route. Simultaneously, severely concentrated chromatin in the nucleus, damaged mitochondrial structure, and impaired energy metabolism were noted, resulting in the loss of ‘LongYan No. 3’ seed vitality and value. Conversely, ‘BaiYan No. 2’ seeds showed a germination rate of 73.33% after 96 h of aging, consistently higher antioxidant enzyme activity during aging, normal embryonic cell shape, and existence of the endoplasmic reticulum.
Conclusions
ROS accumulation and antioxidant enzyme system damage in aged oat seeds, nuclear chromatin condensation, mitochondrial structure damage, nucleic acid metabolism and respiration weakened, oat seed vigor decreased. ‘LongYan No. 3’ seeds were more severely damaged under artificial aging than ‘BaiYan No. 2’ seeds, highlighting their heightened susceptibility to aging effects.
Similar content being viewed by others
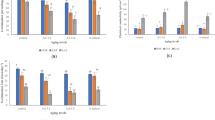
Introduction
Oats, an annual herb belonging to the Gramineae family and pooideae [1], are the sixth most important cereal crop cultivated worldwide and are known for their resistance to soil infertility, salinity, and drought and cold conditions [31, 32]. In the present study, aging treatment significantly increased O2·− production in oat seed cells. In the early stage of aging, SOD activity gradually increased while O2·− production slowed down, indicating enhanced disproportionation of O2·− and its conversion to H2O2 and O2 [33, 34]. Additionally, CAT, which scavenges H2O2 under oxidative stress, exhibited decreased activity as aging progressed, contributing to H2O2 and other ROS accumulation, thereby exacerbating membrane lipid peroxidation, damaging the oat seed’s antioxidant enzyme system, and reducing seed viability [35, 36]. ‘BaiYan No. 2’ seeds consistently exhibited higher antioxidant enzyme activity and greater ROS scavenging ability compared with ‘LongYan No. 3’ seeds. The decrease in ROS levels after reaching their peak is attributed to the antioxidant system’s scavenging effect and damage caused by ROS accumulation to the membrane and mitochondrial structure and function, as well as protein denaturation. This process resulted in an overall decrease in seed metabolism, leading to the gradual decrease in ROS content with seed death. Active oxygen has a great influence on seed vigor, so the production of active oxygen in seeds should be avoided during seed storage. Zinc has been proven effective in reducing ROS generation and defending cells against ROS attack, while Zn deficiency allows higher ROS accumulation causing plant damage [37]. The UV absorption efficiency of nano-ZnO (n-ZnO) makes them highly effective for improving antioxidant enzyme activities, growth and plant performance against abiotic stress [38, 39]. Therefore, in subsequent studies, it is desired to combine aging with n-ZnO pretreatment.
Effect of aging time on the anatomical structures of covered and naked oat seeds
The success of cereal grain germination and initial stages of seedling growth depends on the precise organization of events taking place during this process. This study revealed that high temperature and high humidity conditions accelerated the deterioration of oat seeds. Changes in the moisture content within the seed, driven by environmental humidity, induce horizontal exchange between the seed and environment. The seed hygroscopicity amplifies respiration intensity and hydrolytic enzyme activity (such as protease activity), accelerating nutrient decomposition and seed deterioration [40]. Storage compounds, such as starch, protein, and fat, accumulate primarily in mature seeds in the endosperm and are ready to be mobilized during seed imbibition and subsequent germination [41]. These compounds are hydrolyzed into sugars, amino acids, and fatty acids by the action of amylase, protease, and esterase, respectively. Then, these small molecules produce sugar compounds through deamidation and β-oxidation. Finally, mainly in the seed embryo, sugar compounds through glycolysis and pentose phosphate pathway provide sufficient energy for seeds germination [42,43,44]. Anatomically, oat seeds are similar to the seeds of other graminaceous plants. Paraffin sections revealed that oat seeds consist of a seed coat, embryo, and endosperm, with the embryo concealed within the endosperm [45]. Under ambient storage conditions, these internal tissues form a cohesive structure, with tight binding between various tissues. Following artificial aging under high temperature and high humidity conditions, the embryo cells of ‘LongYan No. 3’ seeds exhibited swelling, with some cells separating from the plasma wall. As aging progressed, the swelling intensified, resulting in larger gaps between cells, leading to a disordered cell arrangement. This change may hinder the physiological pathway of gibberellin into aleurone layer, preventing starch hydrolysis enzymes from entering the endosperm, thereby blocking the nutrient supply pathway and causing a decline in ‘LongYan No. 3’ seed vitality [46]. The aleurone cells are able to perceive gibberellins and induce the synthesis and secretion of hydrolytic enzymes, these cells play a central role to mobilize the storage material of the starchy endosperm and have received more attention than any other grain tissue. Once the aleurone cells have performed their important function, enter in a process of Programmed Cell Death (PCD), which is also activated by gibberellins [47], thus allowing the use of the aleurone cellular contents for seedling growth. ABA is also reported to inhibit seed germination by inhibiting GA3 biosynthesis directly under high temperature [48]. ‘BaiYan No. 2’ seeds showed some swelling in embryonic cells but remained neatly arranged, indicating minimal impact of the aging process on naked oat seed viability. After 8 years of storage, the cells of the aleurone layer of ‘LongYan No. 3’ seeds showed slight fragmentation, whereas those of ‘BaiYan No. 2’ exhibited major breakage, enlarged cell gaps, and severely degraded protein storage vacuoles, with numerous droplet-like structures appearing inside the cells [26]. Scutellar cells undergoing PCD show vacuolization in the cytoplasm and a proactive intramembrane system linking the intracellular secretory pathway to a process of vacuolar cell death [49]. The presence of precursor protease vesicles and autolytic compartments derived from the endoplasmic reticulum [50, 51] and Golgi cisternae [52] are considered as features of plant cell death, resembling morphological features of autophagy in animal cells. Although the role of autophagy in cell death is still subject of discussion [53], both morphological and biochemical evidence suggests that autophagy has a pro-death function either in developmental [54] or pathogen-induced PCD in plants [55]. Under natural storage conditions, the external structures of covered oat protect the caryopsis from various biotic and abiotic stresses, with the lemma playing a protective role. However, the internal structure of ‘BaiYan No. 2’ (naked oat) seeds was affected by artificial aging under high temperature, confirming that this process compromises the external structure protective ability while also highlighting the lower storage resistance of covered oats compared with that of naked oats.
Impact of various aging times on the ultrastructure of seed embryo cells and mitochondria in covered and naked oat seeds
Without artificial aging, normal oat seed vesicles displayed intact organelles and debris-free internal structures, with an intact vesicle membrane, electron-dense material along the membrane, and well-distributed mitochondria and plastids in the cytoplasm. Endoplasmic reticulum and Golgi apparatus structures remained intact, indicating the presence of normal cell metabolism. The euchromatin of oat seed embryo cells was evenly distributed in the nucleus, resulting in an overall lighter cell color. During this period, the DNA double helix structure in the nucleus mainly exists in a relaxed state, and nucleic acids undergo active transcription and synthesis metabolism. A characteristic feature of PCD is that mitochondria show varying degrees of structural alteration. After subjecting oat seeds to high temperature and high humidity conditions, the mitochondrial matrix was diluted and the inner cavity showed vacuolization. This indicates severe mitochondrial damage, resulting in impaired energy metabolism and cellular senescence. Wang et al. revealed that ultrastructural damage in wheat embryo cells became more severe as storage temperature and humidity increased [56]. After aging, the chromatin in the nucleus of oat seed embryo cells became concentrated and the nuclear membrane was dissolved, which indicated weakened nucleic acid metabolism, contributing to reduced seed viability. Cytological changes, particularly in the mitochondrial ultrastructure, occur as seeds deteriorate in response to aging-induced oxidative stress. The mitochondria serve as the primary organelle responsible for producing and being susceptible to free radicals, which act as the energy factory for cellular life activities [57, 58]. When plants are subjected to aging stress, damage to mitochondria, rupture of the mitochondrial membrane or damage to the mitochondrial COX respiratory pathway can result in a blockage of the formation of the transmembrane proton gradient, or an increase in AOX activity can lead to an increase in oxygen consumption in the respiratory electron transport chain but not a significant increase in the transmembrane proton gradient [59], and increased UCP activity leads to a decrease in the transmembrane proton gradient used to drive ATP synthesis [60, 61]. All of these reasons lead to uncoupling of electron transfer from oxidative phosphorylation, making mitochondrial oxidative phosphorylation less efficient. so that the efficiency of mitochondrial oxidative phosphorylation is reduced, respiration is weakened, so that the ATP supply is insufficient during oat seed germination, electron leakage occurs, and free radicals are prompted, leading to a decrease in seed vigor, a decrease in the rate of germination, and t affecting the morphology of the seedling establishment.
Conclusion
With prolonged artificial aging, significant changes were observed in the anatomical structure of oat seeds and the ultrastructure of seed embryo cells and mitochondria. During the artificial aging process, seed embryo cells exhibited swelling and large cell gaps. The nuclear chromatin in aged oat seed embryo cells condensed, and the mitochondrial matrix became diluted and vacuolated, leading to weakened cell metabolism in the oat seed embryo. This weakening ultimately resulted in reduced seed viability, highlighting the close relationship between structure and function. The reduction of the cell membrane’s ability to act as a semipermeable membrane coupled with the accumulation of ROS in the seeds contribute to the destruction of the oat seed antioxidant enzyme system with prolonged aging. The swelling phenomenon in seed embryo cells became more pronounced, disrupting the cellular arrangement and blocking nutrient supply pathways. Additionally, chromatin condensation in the cell nucleus, mitochondrial structure breakage, and impaired normal cell energy metabolism further contribute to the decline in seed vigor, rendering ‘LongYan No. 3’ seeds nonviable. The germination rate and antioxidant enzyme activity of ‘BaiYan No. 2’ seeds were consistently higher than those of ‘LongYan No. 3’ seeds, and the shape of their seed embryo cells remained normal, with visible endoplasmic reticulum. Overall, the deterioration observed in artificially aged ‘LongYan No. 3’ seeds with husks was more pronounced than that in ‘BaiYan No. 2’ naked oats.
Materials and methods
Materials
The test materials were the ‘LongYan No. 3’ variety of covered oats (Avena sativa L.) and ‘BaiYan No. 2’ variety of naked oats (Avena nuda L.). ‘LongYan No. 3’ was a new oat variety bred by crossing Danish black-seed oat 444 as female parent and European black oat Fyris as male parent in 1998. It was selected by Gansu Agricultural University. ‘LongYan No. 3’ has been reported to have superior yield and high nutritional quality, which makes it suitable for cultivation in Tongwei County and similar high-humid mountain area in Gansu Province [62]. ‘BaiYan No. 2’ was bred by pedigree method based on the F4 generation of oat introduced from Canada (numbered B07046). Baicheng Academy of Agricultural Sciences in Jilin Province was selected. These varieties were planted in Huajialing Town, Tongwei County, Gansu Province (altitude: 2457 m; 35°22′46.3″N, 105°0′37.2″E). This location is characterized by a temperate monsoon climate, with an average annual temperature of 3.7℃ and average annual rainfall of 451.1 mm. Seeds harvested in 2020 were stored at room temperature in the forage seed room at Gansu Agricultural University in Lanzhou City. The storage room, ventilated year-round, had an average annual temperature of 10℃–12℃ and relative humidity of 45–64%.
Seed aging treatment
Initial water content of oat seeds was measured and adjusted to 10%. According to the aging method [63], the seeds were subjected to artificial aging at 45 °C for 0, 24, 48, 72, and 96 h under 95% relative humidity. Then, the seeds were dried, restored to their original moisture content, and stored at 4 °C.
Determination of vitality indicators
According to the method in the “Manual of Seedling Evaluation and Seed Vigor Determination Methods” [64], the oat seed germination test was conducted using petri dishes on paper, with counting completed on day 10, after which the fresh weight of seedlings was determined. Each treatment had four replications, and each replication included 50 seeds.
Variables included G10 (number of normal seedlings on day 10), N (total seeds), Nt (number of germinated seeds per day, corresponding to t), t (days of germination), and S (fresh weight of seedlings after 10 days of germination).
Determination of physiological and biochemical indicators
Electrical conductivity (EC) was determined by placing 50 seeds in a 150-ml triangular flask, adding 100 ml of deionized water, shaking the flask until the seeds were completely submerged, and sealing the flask with parafilm. After 24 h (± 15 min) in a 25 °C incubator, the solution conductivity was measured (H198304 [DiST4] [ Italian (HANNA]). Four replicates were used per treatment, with unseeded deionized water used as the control [65].
Malondialdehyde (MDA) content was determined using the thiobarbituric acid (TBA) method. Oat seeds (0.5 g) were homogenized with 2 ml of trichloroacetic acid (TCA), after which 3 ml of TCA was added to the homogenate before further grinding. After centrifugation at 3000 ×g for 10 min, the supernatant was collected as the extraction solution, 2 ml of which was pipetted into a stoppered test tube. Subsequently, 2 ml of TBA solution was added, the mixture was shaken before being placed in a boiling water bath. After 10 min, the solution was removed, allowed to cool, and centrifuged. Finally, optical density (OD) values were measured at 532, 600, and 450 nm. For the control, 2 ml of distilled water was used instead of the extract [65].
Soluble sugar (SS) content was determined using the anthrone method. Oat seeds (0.2 g) were added to a test tube containing 15 ml of distilled water, and the tube was placed in a boiling water bath for 20 min. The mixture was then filtered through centrifugation into a 50-ml volumetric flask. Subsequently, the residue was rinsed, constant volume, and 1 ml of the sample extract was transferred into a 20-ml graduated test tube. After adding 5 ml of anthrone reagent, the solution was vigorously mixed, and the test tube was placed in a boiling water bath for 10 min. After cooling to room temperature, the OD value at 620 nm was measured, zeroing with a blank sample [65].
Soluble protein (SP) content was determined using Thomas Brilliant Blue G-250. Oat seeds (0.5 g) were placed in a mortar, to which 5 ml of phosphate buffer (pH 7.0) was added, and the mixture was ground into a homogenate in an ice bath. Following centrifugation at 4000 ×g for 10 min, 0.1 ml of the sample extract was aspirated, to which 5 ml of Cauldron Brilliant Blue G-250 Reagent was added and mixed thoroughly. The blank control was allowed to stand for 5 min. The OD value at 595 nm was measured using a 1-cm colorimetric cup [65].
Determination of antioxidant enzyme activities
To extract enzymes, seeds embryos (200 mg) were grounded in liquid nitrogen, homogenized in 2 ml phosphate buffer (50 mM, pH 7.0), and then centrifuged at 15 000×g for 20 min at 4℃. The supernatant was used in the antioxidant enzyme assays [66].
The activity of Superoxide dismutase (SOD) (EC 1.15. 1.1) was determined. The 3 ml reaction mixture contained 13 mM methionine, 1.3 µM riboflavin, 63 µM nitroblue tetrazolium (NBT) in 50 mM phosphate buffer (pH 7.8), and 25 µl enzyme extract. The enzyme extract was replaced with phosphate buffer in two controls. The reaction mixtures were incubated in a growth chamber (LRH-250-GII, Ningbo, China) at 25℃ under illumination. Identical tubes that were not illuminated served as blanks. After illumination for 17 min, absorbance was measured at 560 nm.
To measure Catalase (CAT) (EC 1.11.1.6) activity, 50 µl supernatant was mixed with 3.4 ml phosphate buffer (25 mM, pH 7.0, containing 0.1 mM EDTA), and 200 µl H2O2. Enzyme activity was determined by measuring the change in absorbance at 240 nm after 1 min.
Determination of reactive oxygen species
To measure the Superoxide Anion (O2·−), Seed embryos (1 g) were grounded in liquid nitrogen, homogenized in 7 ml phosphate buffer (65 mM, pH 7.8), centrifuged at 10,000 ×g for 10 min. Then, 2 ml supernatant was mixed with 1.5 ml phosphate buffer (65 mM) and 0.5 ml hydroxylamine hydrochloride. Incubated mixture at 25℃ for 20 min, added with 2 ml sulfanilic acid (17 mM) and 2 ml α-naphthylamine (7 mM); the mixture was incubated at 30℃ for 30 min and the absorbance was measured at 530 nm [23].
To measure the Hydrogen Peroxide (H2O2) content, embryos (200 mg) were grounded and homogenized in 2.0 ml cold acetone, centrifuged at 16 000 ×g for 10 min. The supernatant (1 ml) was mixed with 100 µl 10% (w/v) titanium tetrachloride and 200 µl ammonia water, and mixed well and centrifuged at 3000 ×g for 10 min, and the supernatant was discarded. The pellet was dissolved in concentrated sulfuric acid, and then absorbance at 415 nm was recorded. A standard curve was prepared by diluting a 100 µmol/L H2O2 stock solution to 10, 20, 40, 60, 80, 100 µmol/L [23].
Anatomical structure of oat seeds determination
The paraffin sectioning method conventionally was used. Oat seeds was soaked in distilled water for 4 h and then fixed in FAA fixative (formalin: glacial acetic acid:70% ethanol = 1:1:18) for 24 h. Gradient dehydration was performed using various concentrations of ethanol, including 30%, 50%, 70%, 85%, 95%, and anhydrous ethanol. Ethanol and xylene are transparent in steps of 45 min each (anhydrous ethanol: xylene (2:1 v/v), anhydrous ethanol: xylene (1:1), anhydrous ethanol: xylene (1:2), and xylene). The transparent material was immersed in xylene with crushed wax. The wax dip** temperature was raised from 37 °C to 56 °C. It was gradually substituted with pure paraffin wax and left in a warm box at 56 °C overnight. The wax was dipped for 2 days, with three changes of wax. After embedding, the blocks were trimmed and sliced into 10 μm thickness. The slices were baked at 45 °C. The sections were deparaffinized in xylene, rehydrated with an ethanol gradient, and double-stained with saffron-solid green. They were sealed with neutral gum, baked at 40 °C and stored permanently. The samples were observed and photographed under a microscope (Motic Panthera U). Each treatment was repeated five times, and five fields of view were selected for each sample [67].
Ultrastructure observation of embryos and mitochondria
The transmission electron microscopy was employed. Oat seeds were soaked in distilled water for 4 h. The seed embryos were removed with a dissecting needle under a stereomicroscope, pre-fixed with 3% glutaraldehyde and then re-fixed using 1% osmium tetroxide. The embryos were dehydrated step by step in acetone (30%, 50%, 70%, 80%, 90%, 95%, 100%). Ep 812 was embedded, and the semi-thin sections were stained with toluidine blue for optical localization, and sliced on an ultrathin microtome with a diamond knife, and double-stained with uranyl acetate and lead citrate at room temperature. JEM-1400FLASH (Japan Electronics (JEOL)) transmission electron microscope was used to observe the sample sections, and photographs of cells and mitochondria were taken [68].
Statistical analysis
Experimental data were plotted using Microsoft Excel 2010. Two-way analysis of variance (ANOVA) was performed using IBM SPSS Statistics 24.0. Adobe Photoshop CC 2018 was employed for date processing. Differences in all tests were considered statistically significant when p < 0.05. Data are presented as the arithmetic mean (M) ± standard error (SE).
Data availability
The data that support the findings of this study are available from the corresponding author upon request.
Abbreviations
- GP:
-
germination potential
- GR:
-
germination rate
- GI:
-
germination index
- VI:
-
vigor index
- GA3 :
-
Gibberellin
- IAA:
-
Indoleacetic acid
- CTK:
-
Cytokinins
- ABA:
-
Abscisic Acid
- EC:
-
Electrical conductivity
- MDA:
-
Malondialdehyde
- TBA:
-
thiobarbituric acid
- TCA:
-
trichloroacetic acid
- OD:
-
optical density
- SS:
-
Soluble sugar
- SP:
-
Soluble protein
- SOD:
-
Superoxide dismutase
- NBT:
-
nitroblue tetrazolium
- CAT:
-
Catalase
- O2·− :
-
Superoxide Anion
- H2O2 :
-
Hydrogen Peroxide
- ROS:
-
reactive oxygen species
- PCD:
-
Programmed Cell Death
References
Stevens EJ, Armstrong KW, Bezar HJ, Griffin WB, Hampton JB. Fodder oats: an overview. In: Fodder oat. A World Overview. Plant Production and Protection, FOA, Rome, Italy: Series No 33; 2004. pp. 11–18.
**a FS, Chen LL, Yan HF, Sun Y, Li ML, Mao PS. Antioxidant and ultrastructural responses to priming with PEG in aged, ultra-dry oat seed. Seed Sci Technol. 2016;44:556–68. https://doi.org/10.15258/sst.2016.44.3.12.
Yi K, Yue J, Yang S, Jiang Y, Hong L, Zeng H, Li M. Germination of aged oat seeds associated with changes in antioxidant enzyme activity and storage compounds mobilization. Physiol Plant. 2023;175:e14020–14020. https://doi.org/10.1111/ppl.14020.
Zhang B, Ren CZ. Advances in Oat Genomic Research and molecular breeding. Chin Bull Bot. 2022;57:785–91. https://doi.org/10.11983/CBB22182.
Deepa GT, Chetti MB, Khetagoudar MC, Adavirao GM. Influence of vacuum packaging on seed quality and mineral contents in Chilli (Capsicum annuum L). J Food Sci Technol. 2013;50:153–8. https://doi.org/10.1007/s13197-011-0241-3.
Mc Donald MB. Seed deterioration: physiology, repair and assessment. 1999.
Niu Z, Jiang H, Zhang R, Xu LY, Lv G, Ch, Zhang ZX, Tian X. Differences in antioxidant physiological responsesof Heteromorphic Cenchrus pauci florus seeds during Artificial Aging. Chin J Grassland:1–6[2023-10-12].
Wood PJ. Cereal β-glucans in diet and health. J Cereal Sci. 2007;46:230–8. https://doi.org/10.1016/j.jcs.2007.06.012.
Brindzová L, Čertík M, Rapta P, Zalibera M, Mikulajová A, Takácsová M. Antioxidant activity, β-glucan and lipid contents of oat varieties. Czech J Food Sci. 2008;26:163. https://doi.org/10.17221/2564-cjfs.
Lehtinen P, Kiiliäinen K, Lehtomäki I, Laakso S. Effect of heat treatment on lipid stability in processed oats. J Cereal Sci. 2003;37:215–21. https://doi.org/10.1006/jcrs.2002.0496.
Ruiz M, Martı́n I, de la Cuadra C. Cereal seed viability after 10 years of storage in active and base germplasm collections. Field Crops Res. 1999;64:229–36. https://doi.org/10.1016/s0378-4290(99)00044-1.
Jiang XC, Zhou SQ. Research Progress on Molecular mechanisms of seed Vigor or anti-aging ability. Life Sci Res. 2021;25:406–16. https://doi.org/10.16605/j.cnki.1007-7847.2021.08.0193.
Heiniö RL, Oksman-Caldentey K, Latva‐Kala MK, Lehtinen P, Poutanen K. Effect of drying treatment conditions on sensory profile of germinated oat. Cereal Chem. 2001;78:707–14. https://doi.org/10.1094/CCHEM.2001.78.6.707.
Murthy UN, Kumar PP, Sun WQ. Mechanisms of seed ageing under different storage conditions for Vigna radiata (L.) Wilczek: lipid peroxidation, sugar hydrolysis, Maillard reactions and their relationship to glass state transition. J Exp Bot. 2003;54:1057–67. https://doi.org/10.1093/jxb/erg092.
Fu YB, Ahmed Z, Yang H, Horbach C. TUNEL assay and DAPI staining revealed few alterations of cellular morphology in naturally and artificially aged seeds of cultivated flax. Plants. 2018;7:34. https://doi.org/10.3390/plants7020034.
Rajjou L, Lovigny Y, Groot SP, Belghazi M, Job C, Job D. Proteome-wide characterization of seed aging in Arabidopsis: a comparison between artificial and natural aging protocols. Plant Physiol. 2008;148:620–41. https://doi.org/10.1104/pp.108.123141.
Giurizatto MIK, Ferrarese-Filho O, Ferrarese MDLL, Robaina AD, Gonçalves MC, Cardoso CAL. α-Tocopherol levels in natural and artificial aging of soybean seeds. Acta Scientiarum Agron 20123; 4:339–43. https://doi.org/10.4025/actasciagron.v34i3.12660.
Priestley DA, Leopold AC. Lipid changes during natural aging of soybean seeds. Physiol Plant. 1983;59:467–70. https://doi.org/10.1111/j.1399-3054.1983.tb04231.x.
Lin Q, Wang W, Ren Y, Jiang Y, Sun A, Qian Y, Wan J. Genetic dissection of seed storability using two different populations with a same parent rice cultivar N22. Breed Sci. 2015;65:411–9. https://doi.org/10.1270/jsbbs.65.411.
**a F, Cheng H, Chen L, Zhu H, Mao P, Wang M. Influence of exogenous ascorbic acid and glutathione priming on mitochondrial structural and functional systems to alleviate aging damage in oat seeds. BMC Plant Biol. 2020;20:1–11. https://doi.org/10.1186/s12870-020-2321-x.
Huang Y, Liu H, Zhao GQ, Wang QY, Luo JM, Yao RR. Effects of Natural Aging and Artificial Aging on Germination characteristics and genetic Integrity of Oat Seeds. Acta Agrestia Sinica. 2022. https://doi.org/10.11733/j.issn.1007-0435.2022.08.017. 30:2066.
**n X, Tian Q, Yin G, Chen X, Zhang J, Ng S, Lu X. Reduced mitochondrial and ascorbate-glutathione activity after artificial ageing in soybean seed. J Plant Physiol. 2014;171:140–7. https://doi.org/10.1016/j.jplph.2013.09.016.
Kong L, Huo H, Mao P. Antioxidant response and related gene expression in aged oat seed. Front Plant Sci. 2015;6:158. https://doi.org/10.3389/fpls.2015.00158.
Lazar SL, Mira S, Pamfil D, Martinez-Laborde JB. Germination and electrical conductivity tests on artificially aged seed lots of 2 wall-rocket species. Turkish J Agric Forestry. 2014;38:857–64. https://doi.org/10.3906/tar-1402-76.
Goel A, Goel AK, Sheoran IS. Changes in oxidative stress enzymes during artificial ageing in cotton (Gossypium hirsutum L.) seeds. J Plant Physiol. 2003;160:1093–100. https://doi.org/10.1078/0176-1617-00881.
Yao RR, Liu H, Zhao GQ, Wang JL, Wang QY, Dong K, Zhang R. Effects of seed storage time on seed germination and cytological structure of covered oats and naked oats. Acta Prataculturae Sinica. 2024;33:154. https://doi.org/10.11686/cyxb2023136.
Ebone LA, Caverzan A, Chavarria G. Physiologic alterations in orthodox seeds due to deterioration processes. Plant Physiol Biochem. 2019;145:34–42. https://doi.org/10.1016/j.plaphy.2019.10.028.
Fu YB, Ahmed Z, Diederichsen A. Towards a better monitoring of seed ageing under ex situ seed conservation. Conserv Physiol. 2015;3:cov026. https://doi.org/10.1093/conphys/cov026.
Suresh A, Shah N, Kotecha M, Robin P. Evaluation of biochemical and physiological changes in seeds of Jatropha curcas L under natural aging, accelerated aging and saturated salt accelerated aging. Sci Hort. 2019;255:21–9. https://doi.org/10.1016/j.scienta.2019.05.014.
Wang P, Li D, Wang LJ. Drying damage on physiological properties of rice seed associated with ultrastructure changes. Int J Food Eng. 2017;13:20170096. https://doi.org/10.1515/ijfe-2017-0096.
Bailly C, Leymarie J, Lehner A, Rousseau S, Côme D, Corbineau F. Catalase activity and expression in develo** sunflower seeds as related to drying. J Exp Bot. 2004;55:475–83. https://doi.org/10.1093/jxb/erh050.
Ahmad MA, Javed R, Adeel M, Rizwan M, Yang Y. PEG 6000-stimulated drought stress improves the attributes of in vitro growth, steviol glycosides production, and antioxidant activities in Stevia rebaudiana Bertoni. Plants. 2020;9(11):1552. https://doi.org/10.3390/plants9111552.
Erdal S, Aydın M, Genisel M, Taspınar MS, Dumlupinar R, Kaya O, Gorcek Z. Effects of salicylic acid on wheat salt sensitivity. Afr J Biotechnol. 2011;10:5713–8. https://doi.org/10.5897/AJB10.1550.
Yin G, **n X, Song C, Chen X, Zhang J, Wu S, Lu X. Activity levels and expression of antioxidant enzymes in the ascorbate–glutathione cycle in artificially aged rice seed. Plant Physiol Biochem. 2014;80:1–9. https://doi.org/10.1016/j.plaphy.2014.03.006.
Bailly C, Bogatek-Leszczynska R, Côme D, Corbineau F. Changes in activities of antioxidant enzymes and lipoxygenase during growth of sunflower seedlings from seeds of different vigour. Seed Sci Res. 2002;12:47–55. https://doi.org/10.1079/ssr200197.
Kibinza S, Bazin J, Bailly C, Farrant JM, Corbineau F, El-Maarouf-Bouteau H. Catalase is a key enzyme in seed recovery from ageing during priming. Plant Sci. 2011;181:309–15. https://doi.org/10.1016/j.plantsci.2011.06.003.
Sturikova H, Krystofova O, Huska D, Adam V. Zinc, zinc nanoparticles and plants. J Hazard Mater. 2018;349:101–10. https://doi.org/10.1016/j.jhazmat.2018.01.040.
Caldelas C, Weiss DJ. Zinc homeostasis and isotopic fractionation in plants: a review. Plant Soil. 2017;411:17–46. https://doi.org/10.1007/s11104-016-3146-0.
Munir T, Rizwan M, Kashif M, et al. Effect of zinc oxide nanoparticles on the growth and zn uptake in wheat (triticumaestivum L.) by seed priming method[J]. Volume 13. Digest Journal of Nanomaterials & Biostructures (DJNB); 2018. 1.
Okamoto K, Murai T, Eguchi G, Okamoto M, Akazawa T. Enzymic mechanism of starch breakdown in germinating rice seeds: 11. Ultrastructural changes in scutellar epithelium. Plant Physiol. 1982;70:905–11. https://doi.org/10.1104/pp.70.3.905.
Bewley JD. Seed germination and dormancy. The plant cell, 1997, 9(7): 1055. https://doi.org/10.1105/tpc.9.7.1055.
Graham IA. Seed storage oil mobilization. Annu Rev Plant Biol. 2008;59:115–42. https://doi.org/10.1146/annurev.arplant.59.032607.092938.
Theodoulou FL, Eastmond PJ. Seed storage oil catabolism: a story of give and take. Curr Opin Plant Biol. 2012;15(3):322–8. https://doi.org/10.1016/j.pbi.2012.03.017.
Zeeman SC, Kossmann J, Smith AM. Starch: its metabolism, evolution, and biotechnological modification in plants. Annu Rev Plant Biol. 2010;61:209–34. https://doi.org/10.1146/annurev-arplant-042809-112301.
White EM. Structure and development of oats. oat crop: Prod Utilization. 1995;88–119. https://doi.org/10.1007/978-94-011-0015-1-4.
Zheng Q, Wang HN, Chang H, Zhang JW, Nan M, Wang M, Zhuang ZY. Effects of freezing injury on the germination characteristics and inner ultrastructure of maize hybridize seeds. J Gansu Agricultural Univ. 2010;45:35–9. https://doi.org/10.1016/j.plaphy.2010.08.016.
Bethke PC, Lonsdale JE, Fath A, Jones RL. Hormonally regulated programmed cell death in barley aleurone cells. Plant Cell. 1999;11(6):1033–45. https://doi.org/10.1105/tpc.11.6.1033.
Toh S, Imamura A, Watanabe A, Nakabayashi K, Okamoto M, Jikumaru Y, Hanada A, Aso Y, Ishiyama K. High temperature-induced abscisic acid biosynthesis and its role in the inhibition of gibberellin action in Arabidopsis seeds. Plant Physiol. 2008;146(3):1368–85. https://doi.org/10.1104/pp.107.113738.
Van Doorn WG, Beers EP, Dangl JL, Franklin-Tong VE, Gallois P, Hara-Nishimura I. Morphological classification of plant cell deaths. Cell Death Differ. 2011;18(8):1241–6. https://doi.org/10.1038/cdd.2011.36.
Toyooka K, Okamoto T, Minamikawa T. Mass transport of proform of a KDEL-tailed cysteine proteinase (SH-EP) to protein storage vacuoles by endoplasmic reticulum–derived vesicle is involved in protein mobilization in germinating seeds. J Cell Biol. 2000;148(3):453–64. https://doi.org/10.1083/jcb.148.3.453.
Greenwood JS, Helm M, Gietl C. Ricinosomes and endosperm transfer cell structure in programmed cell death of the nucellus during Ricinus seed development. Proceedings of the National Academy of Sciences, 2005, 102(6): 2238–2243. https://doi.org/10.1073/pnas.0409429102.
Filonova LH, Bozhkov PV, Brukhin VB, Daniel G, Zhivotovsky B, Arnold SV. Two waves of programmed cell death occur during formation and development of somatic embryos in the gymnosperm, Norway spruce. J Cell Sci. 2000;113(24):4399–411. https://doi.org/10.1242/jcs.113.24.4399.
Kroemer G, Levine B. Autophagic cell death: the story of a misnomer. Nat Rev Mol Cell Biol. 2008;9(12):1004–10. https://doi.org/10.1038/nrm2529.
Bozhkov PV, Filonova LH, Suarez MF. 4-Programmed cell death in plant embryogenesis. Curr Top Dev Biol. 2005;67:135–79. https://doi.org/10.1016/S0070-2153(05)67004-4.
Liu Y, Schiff M, Czymmek K, Tallóczy Z, Levine B, Dinesh-Kumar SP. Autophagy regulates programmed cell death during the plant innate immune response. Cell. 2005;121(4):567–77. https://doi.org/10.1016/j.cell.2005.03.007.
Wang RL, Zhang LL, Cao ZS, Wang C. Ultra-structural changes in wheat embryo cell and aging mechanism under micro- environment storage conditions. J Chin Cereals Oils Association. 2014;29:77–82. CNKI: SUN: ZLYX.0.2014-10-017.
**a F, Chen L, Sun Y, Mao P. Relationships between ultrastructure of embryo cells and biochemical variations during ageing of oat (Avena sativa L.) seeds with different moisture content. Acta Physiol Plant. 2015;37:1–11. https://doi.org/10.1007/s11738-015-1825-8.
Zhang LL, Wang RL, Liu L, Mu Y. Variation of mitochondrial ultrastructure and antioxidative enzymatic activity in wheat cell under micro-environmental conditions. Mod Food Sci Technol. 2014;30:81–6. https://doi.org/10.13982/j.mfst.1673-9078.2014.03.023.
Jacoby RP, Taylor NL, Millar AH. The role of mitochondrial respiration in salinity tolerance. Trends Plant Sci. 2011;16(11):614–23. https://doi.org/10.1016/j.tplants.2011.08.002.
Jarmuszkiewicz W, Behrendt M, Navet R, Sluse FE. Uncoupling protein and alternative oxidase of Dictyostelium Discoideum: occurrence, properties and protein expression during vegetative life and starvation-induced early development. FEBS Lett. 2002;532(3):459–64. https://doi.org/10.1016/S0014-5793(02)03734-1.
Woyda-Ploszczyca A, Koziel A, Antos-Krzeminska N, Jarmuszkiewicz W. Impact of oxidative stress on Acanthamoeba castellanii mitochondrial bioenergetics depends on cell growth stage. J Bioenerg Biomembr. 2011;43:217–25. https://doi.org/10.1007/s10863-011-9351-x.
Ju ZL, Zhao GQ, Chai JK, Jia Zf, Liang GL. Comprehensive evaluation of nutritional value and silage fermentation quality of different oatvarieties in central Gansu Province. Acta Prataculturae Sinica. 2019;28(09):77–86. https://doi.org/10.11686/cyxb2018542.
Zheng XY, Ren ZS, Zhen GH. Discussion on the method of determining seed vigor - III. Artificial accelerated aging method. Seed. 1982;31–4. https://doi.org/10.16590/j.cnki.1001-4705.1982.04.024.
International Seed Testing Association (ISTA). Manual of methods for seedling assessment and seed viability determination. Bei**g: Bei**g Agricultural University; 1987. pp. 1–12.
Zhang ZA, Chen ZY. Experimental techniques in plant physiology. Chang Chun: Jilin University; 2008. pp. 171–286.
Beauchamp C, Fridovich I. Superoxide dismutase: improved assays and an assay applicable to acrylamide gels. Anal Biochem. 1971;44:276–87. https://doi.org/10.1016/0003-2697(71)90370-8.
Liu W, Xu X, Wang X. Unique Morphology of Sarcobatus baileyi Male Inflorescence and Its Botanical Implications. Plants. 2023. https://doi.org/10.3390/plants12091917. 12:1917.
** B, Wang L, Wang J, Jiang KZ, Wang Y, Jiang XX, Teng NJ. The effect of experimental warming on leaf functional traits, leaf structure and leaf biochemistry in Arabidopsis thaliana. BMC Plant Biol. 2011;11:1–10. https://doi.org/10.1186/1471-2229-11-35.
Acknowledgements
The authors are thankful to Teachers, such as Jiangang Chen, Director of the Laboratory, for providing the required laboratory facilities, and we are thankful to graduate student Yuan Ma, Bin Han, Jiahui Dong, Gansu Agricultural University, for their continuous support to carry out this work.
Funding
This research was funded by China National Natural Science Foundation (32360344), Key Laboratory of Grassland Ecosystem Ministry of Education Project (KLGE2022-16), National Key Research and Development Program Foundation (2022YFD1100502), and Gansu Agricultural University Youth Tutor Fund (GAU-QDFC-2023-01), Fund by China scholarship council.
Author information
Authors and Affiliations
Contributions
Y.-R.R., L.H., S.-S.L., W.-J.L., Z.-G.Q., and Z.-X.R. conceived and designed the project. Y.-R.R. performed the experiments. Y.-R.R. and L.H. analyzed the data and wrote the paper.
Corresponding author
Ethics declarations
Ethics approval and consent to participate
In this study, Avena sativa seeds were obtained and collected from Gansu Agricultural University. Professor Huan Liu has gained the permission from Gansu Agricultural University to perform a breeding trial. Avena nuda seeds were collected with permission by Baicheng Academy of Agricultural Sciences in Jilin Province in accordance with institutional and national guidelines.
Consent for publication
Not applicable.
Competing interests
The authors declare no competing interests.
Additional information
Publisher’s Note
Springer Nature remains neutral with regard to jurisdictional claims in published maps and institutional affiliations.
Rights and permissions
Open Access This article is licensed under a Creative Commons Attribution 4.0 International License, which permits use, sharing, adaptation, distribution and reproduction in any medium or format, as long as you give appropriate credit to the original author(s) and the source, provide a link to the Creative Commons licence, and indicate if changes were made. The images or other third party material in this article are included in the article’s Creative Commons licence, unless indicated otherwise in a credit line to the material. If material is not included in the article’s Creative Commons licence and your intended use is not permitted by statutory regulation or exceeds the permitted use, you will need to obtain permission directly from the copyright holder. To view a copy of this licence, visit http://creativecommons.org/licenses/by/4.0/. The Creative Commons Public Domain Dedication waiver (http://creativecommons.org/publicdomain/zero/1.0/) applies to the data made available in this article, unless otherwise stated in a credit line to the data.
About this article
Cite this article
Yao, R., Liu, H., Wang, J. et al. Cytological structures and physiological and biochemical characteristics of covered oat (Avena sativa L.) and naked oat (Avena nuda L.) seeds during high-temperature artificial aging. BMC Plant Biol 24, 530 (2024). https://doi.org/10.1186/s12870-024-05221-2
Received:
Accepted:
Published:
DOI: https://doi.org/10.1186/s12870-024-05221-2