Abstract
To obtain the heat power or the power absorbed by nanoparticles under sunlight, we presented a numerical method that by obtaining the absorption cross section of nanoparticles which is exposed to plane-wave incident light and then multiplying it in the solar spectrum, the absorption spectrum of nanoparticles under solar radiation is obtained and by integrating the absorption spectrum, the total power absorbed by the nanoparticles under the sunlight is obtained. We applied the above method to obtain the heat power of gold, silver, aluminum, and copper nanoparticles with the same volume of metal and in three different spherical, cubic, and rod morphologies. The results showed that for gold nanoparticles, the cubic structure produces the highest heat power and about 1.7 times the spherical structure; for silver nanoparticles, the rod structure with aspect ratio 2.6:1 produces the highest heat power and about 1.8 times the spherical structure; for aluminum nanoparticles, the rod structure with aspect ratio 2.6:1 produces the highest heat power and about 2.2 times the spherical structure; and for copper nanoparticles, the cubic structure produces the highest heat power and about 1.3 times the spherical structure. Also, we have investigated the effect of nanoparticle aggregation on absorbed power under sunlight. The results of this research can be used for solar thermal applications with the help of plasmonic nanoparticles.




































Similar content being viewed by others
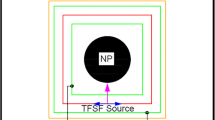
Data Availability
The datasets generated during and/or analyzed during the current study are available from the corresponding author on reasonable request.
Code Availability
Software application or custom code generated during and/or analyzed during the current study are available from the corresponding author on reasonable request.
References
Neumann O, Urban AS, Day J, Lal S, Nordlander P, Halas NJ (2013) Solar vapor generation enabled by nanoparticles. ACSNANOA 7(1):42–49. https://doi.org/10.1021/nn304948h
Zhou L, Tan Y, Ji D, Zhu B, Zhang P, Xu J, Gan Q, Yu Z, Zhu J (2016) Self-assembly of highly efficient, broadband plasmonic absorbers for solar steam generation. Sci Adv 2(4 e1501227):1–8. https://doi.org/10.1126/sciadv.1501227
Zazoum B, El Hassan M, Jendoubi A (2020) Solar optical fiber daylighting system with an IR filter: experimental and modeling studies. Energy Rep 6(Supplement 9):903–908. https://doi.org/10.1016/j.egyr.2020.11.111
Li L, Wang J, Yang Z, Lou G (2018) An optical fiber daylighting system with large Fresnel lens. Energy Procedia 152:342–347. https://doi.org/10.1016/j.egypro.2018.09.146
Huang X, El-Sayed MA (2010) Gold nanoparticles: optical properties and implementations in cancer diagnosis and photothermal therapy. Adv Res 1:13–28. https://doi.org/10.1016/j.jare.2010.02.002
Vines JB, Yoon JH, Ryu NE, Lim DJ, Park H (2019) Gold nanoparticles for photothermal cancer therapy. Front Chem 7(167):1–16. https://doi.org/10.3389/fchem.2019.00167
Grosges T, Barchiesi D (2018) Gold nanoparticles as a photothermal agent in cancer therapy: the thermal ablation characteristic length. Molecules 23(1316):1–13. https://doi.org/10.3390/molecules23061316
Gharatape A, Davaran S, Salehi R, Hamishehkar H (2016) Engineered gold nanoparticles for photothermal cancer therapy and bacteria killing. RSC Adv 6:111482–111516. https://doi.org/10.1039/c6ra18760a
Jong WHD, Borm PJ (2008) Drug delivery and nanoparticles: applications and hazards. Int J Nanomed 3(2):133–149
Yu X, Trase I, Ren M, Duval K, Guo X, Chen Z (2016) Design of nanoparticle-based carriers for targeted drug delivery. J Nanomater 1087250:1–15. https://doi.org/10.1155/2016/1087250
Cheng H, Wang Ch, Xu Zh, Lin H, Zhang Ch (2015) Gold nanoparticle-enhanced near infrared fluorescent nanocomposites for targeted bio-imaging. RSC Adv 5:20–26. https://doi.org/10.1039/c4ra12066c
Selvan ST, Tan TTY, Yi DK, Jan NR (2010) Functional and multifunctional nanoparticles for bioimaging and biosensing. Langmuir 26(14):11631–11641. https://doi.org/10.1021/la903512m
Walsh T, Lee J, Park K (2015) Aser-assisted photothermal heating of a plasmonic nanoparticle-suspended droplet in a microchannel. Analyst 140:1535–1542. https://doi.org/10.1039/c4an01750a
Cheong K, Yi DK, Lee JG, Park JM, Kim MJ, Edel JB, Ko Ch (2008) Gold nanoparticles for one step DNA extraction and real time PCR of pathogens in a single chamber. Lab Chip 8:810–813. https://doi.org/10.1039/b717382b
Kim J, Kim H, Park JH, Jon S (2017) Gold Nanorod-based Photo-PCR System for One-Step, rapid detection of bacteria. Nanotheranostics 1:178–185. https://doi.org/10.7150/ntno.18720
Roche PhJR, Najih M, Lee SS, Beitel LK, Paliouras MCM, Kirk AG, Trifiro MA (2017) Real time plasmonic qPCR: how fast is ultra-fast? 30 cycles in 54 seconds. Analyst 142:1746–1755. https://doi.org/10.1039/c7an00304h
Ishii S, Sugavaneshwar RP, Chen K, Dao TD, Nagao T (2016) Solar water heating and vaporization with silicon nanoparticles at mie resonances. Opt Mater Express 6(2):640–648. https://doi.org/10.1364/ome.6.000640
Taylor RA, Phelan PE, Otanicar TP, Walker CA, Nguyen M, Trimble S, Prasher R (2011) Applicability of nanofluids in high flux solar collectors. Renew Sustain Energy 3(023104):1–8. https://doi.org/10.1063/1.3571565
Neumanna O, Feronti C, Neumann AD, Dong A, Schell K, Lue B, Kim E, Quinn M, Thompsone Sh, Grady N, Nordlander P, Oden M, Halas NJ (2013) Compact solar autoclave based on steam generation using broadband light-harvesting nanoparticles. PNAS 110(29):11677–11681. https://doi.org/10.1073/pnas.1310131110
Chen CJ, Chen DH (2013) Preparation and near-infrared photothermal conversion property of cesium tungsten oxide nanoparticles. Nanoscale Res Lett 8(57):1–8. https://doi.org/10.1186/1556-276x-8-57
Mercatelli L, Sani E, Zaccanti G, Martelli F, Ninni PD, Barison SA, Pagura C, Agresti F, Jafrancesco D (2011) Absorption and scattering properties of carbon nanohorn-based nanofluids for direct sunlight absorbers. Nanoscale Res Lett 6(282):1–9. https://doi.org/10.1186/1556-276x-6-282
Baffou G, Quidant R, García de Abajo FJ (2010) Nanoscale control of optical heating in complex plasmonic systems. ACSNANO 4(2):709–716. https://doi.org/10.1021/nn901144d
Sassaroli E, Li KCP, Neill BEO (2009) Numerical investigation of heating of a gold nanoparticle and the surrounding microenvironment by nanosecond laser pulses for nanomedicine applications. Phys Med Biol 54:5541–5560. https://doi.org/10.1088/0031-9155/54/18/013
Baffou G, Quidant R, Girard C (2009) Heat generation in plasmonic nanostructures: influence of morphology. Appl Phys Lett 94:153109. https://doi.org/10.1063/1.3116645
Ni Y, Kan C, Gao Q, Wei J, Xu H, Wang Ch (2016) Heat generation and stability of a plasmonic nanogold system. J Phys D: Appl Phys 49:055302. https://doi.org/10.1088/0022-3727/49/5/055302
Kelly KL, Coronado E, Zhao LL, Schatz GC (2003) The optical properties of metal nanoparticles: the influence of size, shape, and dielectric environment. J Phys Chem B 107:668–677. https://doi.org/10.1021/jp026731y
Asgharian A, Yadipour R, Kiani G, Baghban H (2020) Heat generation and light transmission in porous plasmonic nanostructures. J Nanophoton 14(1):016007. https://doi.org/10.1117/1.JNP.14.016007
Govorov AO, Zhang W, Skeini T, Richardson H, Lee J, Kotov NA (2006) Gold nanoparticle ensembles as heaters and actuators: melting and collective plasmon resonances. Nanoscale Res Lett 1:84–90. https://doi.org/10.1007/s11671-006-9015-7
Govorov AO, Richardson HH (2007) Generating heat with metal nanoparticles. Nanotoday 2(1):30–38
Ishii S, Sugavaneshwar RP, Tadaaki N (2016) Titanium nitride nanoparticles as plasmonic solar heat transducers. J Phys Chem C 120(4):2343–2348. https://doi.org/10.1021/acs.jpcc.5b09604
Finger F, Welter K, Urbain F, Smirnov V (2020) Photoelectrochemical water splitting using adapted silicon based multi-junction solar cell structures: development of solar cells and catalysts, upscaling of combined photovoltaic-electrochemical devices and performance stability. Z Phys Chem 234(6):1055–1095. https://doi.org/10.1515/zpch-2019-1453
Sebastian N (2015) Limiting approach to generalized gamma bessel model via fractional calculus and its applications in various disciplines. Axioms 4:385–399. https://doi.org/10.3390/axioms4030385
Würfel P, Finkbeiner S, Daub E (1995) Generalized Planck’s radiation law for luminescence via indirect transitions. Appl Phys A 60:67–70. https://doi.org/10.1007/BF01577615
Planck M (1901) Distribution of energy in the spectrum. Ann Phys 4:553–563
Zhou L, Tan Y, Wang J et al (2016) 3D self-assembly of aluminium nanoparticles for plasmon-enhanced solar desalination. Nat Photon 10:393–398. https://doi.org/10.1038/nphoton.2016.75
Balela MD, Amores KL (2015) Formation of oxidation-stable copper nanoparticles in water. Adv Mater Res 1131:255–259. https://doi.org/10.4028/www.scientific.net/AMR.1131.255
Chang SJ, Tung CA, Chen BW, Chou YC, Li CC (2013) Synthesis of non-oxidative copper nanoparticles. RSC Adv 3:24005–24008. https://doi.org/10.1039/C3RA44768E
Funding
The authors declare that no funds, grants, or other support were received during the preparation of this manuscript.
Author information
Authors and Affiliations
Contributions
All authors contributed to the study conception and design. All authors read and approved the final manuscript and consent for publication data and images.
Corresponding author
Ethics declarations
Ethics Approval
Not applicable.
Consent to Participate
Not applicable.
Consent for Publication
Not applicable.
Competing Interests
The authors declare no competing interests.
Additional information
Publisher's Note
Springer Nature remains neutral with regard to jurisdictional claims in published maps and institutional affiliations.
Rights and permissions
About this article
Cite this article
Asgharian, A., Yadipour, R., Kiani, G. et al. Proposing a Numerical Method to Calculate the Absorbed Power of Plasmonic Nanoparticles (Au, Ag, Al, and Cu) with Different Morphologies Under Solar Irradiance. Plasmonics 17, 1527–1547 (2022). https://doi.org/10.1007/s11468-022-01641-8
Received:
Accepted:
Published:
Issue Date:
DOI: https://doi.org/10.1007/s11468-022-01641-8