Abstract
The direct ethanol fuel cell research for high efficiency and portable applications aims to enhance the electrochemical activity and lifetime of the electrocatalyst. Nonetheless, the major portion of electrocatalyst consists of support, 60 to 80% m/m. The physical–chemical properties of support material can directly influence electrochemical activity. This study compares different carbon allotropes as supports of Pt3Sn electrocatalyst, carbon black (C) and graphite (Gr) by commercial acquisition, and carbon spheres (CS) and graphene (G) prepared by our research group using hydrothermal synthesis and thermal expansion methods, respectively. Carbon black is the widely used support. Adopting it as a reference, in acid electrolyte, the forward oxidation current of CV decreases 97% (Pt3Sn/CS), 62% (Pt3Sn/G), and 78% (Pt3Sn/Gr). Nonetheless, in chronoamperometry (CA), Pt3Sn/G exhibited an increase of 48% in the final current compared with Pt3Sn/C, while Pt3Sn/CS current decreased 94% and Pt3Sn/Gr 67%. In alkaline electrolyte, Pt3Sn/G excels further among other materials once the ethanol oxidation current increases 60% in cyclic voltammetry (CV) and final current 36% higher by CA. The other allotropes decreased 99% (Pt3Sn/CS) and 54% (Pt3Sn/Gr) in cyclic voltammetry and exhibited a CA final current 84% (Pt3Sn/CS) and 12% (Pt3Sn/Gr) lower than Pt3Sn/C. Pt3Sn/G has high electrical conductivity and a large surface area which can contribute to enhancement of ethanol oxidation in activity sites. Therefore, the allotrope structure directly influences the electrochemical activity and that can be used to enhance the ethanol oxidation by electrocatalyst, where graphene supports the best improvement.










Similar content being viewed by others
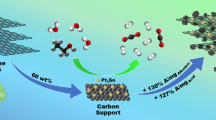
References
Geddes CC, Nieves IU, Ingram LO (2011) Advances in ethanol production. Curr Opin Biotechnol 22:312–319. https://doi.org/10.1016/j.copbio.2011.04.012
Latif MN, Wan Isahak WNR, Samsuri A et al (2023) Recent advances in the technologies and catalytic processes of ethanol production. Catalysts 13:1093. https://doi.org/10.3390/catal13071093
McNicol B (2001) Fuel cells for road transportation purposes   yes or no? J Power Sources 100:47–59. https://doi.org/10.1016/S0378-7753(01)00882-5
Raj T, Chandrasekhar K, Naresh Kumar A et al (2022) Recent advances in commercial biorefineries for lignocellulosic ethanol production: Current status, challenges and future perspectives. Bioresour Technol 344. https://doi.org/10.1016/j.biortech.2021.126292
da Silva TN, Coradi PC, dos Santos BN et al (2023) Effects of corn drying and storage conditions on flour, starch, feed, and ethanol production: A review. J Food Sci Technol 60:2337–2349. https://doi.org/10.1007/s13197-022-05549-3
Akhairi MAF, Kamarudin SK (2016) Catalysts in direct ethanol fuel cell (DEFC): An overview. Int J Hydrogen Energy 41:4214–4228. https://doi.org/10.1016/j.ijhydene.2015.12.145
Wang J, Wasmus S, Savinell RF (1995) Evaluation of ethanol, 1-propanol, and 2-propanol in a direct oxidation polymer-electrolyte fuel cell: A real-time mass spectrometry study. J Electrochem Soc 142:4218–4224. https://doi.org/10.1149/1.2048487
Camara GA, Iwasita T (2005) Parallel pathways of ethanol oxidation: The effect of ethanol concentration. J Electroanal Chem 578:315–321. https://doi.org/10.1016/j.jelechem.2005.01.013
Marinkovic NS, Li M, Adzic RR (2019) Pt-based catalysts for electrochemical oxidation of ethanol. Top Curr Chem 377:11. https://doi.org/10.1007/s41061-019-0236-5
Zheng Y, Wan X, Cheng X et al (2020) Advanced catalytic materials for ethanol oxidation in direct ethanol fuel cells. Catalysts 10:166. https://doi.org/10.3390/catal10020166
Frelink T, Visscher W, van Veen JAR (1995) On the role of Ru and Sn as promotors of methanol electro-oxidation over Pt. Surf Sci 335:353–360. https://doi.org/10.1016/0039-6028(95)00412-2
Watanabe M, Motoo S (1975) Electrocatalysis by ad-atoms. J Electroanal Chem Interfacial Electrochem 60:275–283. https://doi.org/10.1016/S0022-0728(75)80262-2
Antolini E (2007) Catalysts for direct ethanol fuel cells. J Power Sources 170:1–12. https://doi.org/10.1016/j.jpowsour.2007.04.009
Demirci UB (2007) Theoretical means for searching bimetallic alloys as anode electrocatalysts for direct liquid-feed fuel cells. J Power Sources 173:11–18. https://doi.org/10.1016/j.jpowsour.2007.04.069
Singh BK, Mahapatra SS (2023) Performance study of palladium modified platinum anode in direct ethanol fuel cells: A green power source. J Indian Chem Soc 100. https://doi.org/10.1016/j.jics.2022.100876
BayrakçekenYurtcan A, Daş E (2018) Chemically synthesized reduced graphene oxide-carbon black based hybrid catalysts for PEM fuel cells. Int J Hydrogen Energy 43:18691–18701. https://doi.org/10.1016/j.ijhydene.2018.06.186
Colmati F, Antolini E, Gonzalez ER (2006) Effect of temperature on the mechanism of ethanol oxidation on carbon supported Pt, PtRu and Pt3Sn electrocatalysts. J Power Sources 157:98–103. https://doi.org/10.1016/j.jpowsour.2005.07.087
Li W, Zhou W, Li H et al (2004) Nano-stuctured Pt–Fe/C as cathode catalyst in direct methanol fuel cell. Electrochim Acta 49:1045–1055. https://doi.org/10.1016/j.electacta.2003.10.015
Wang Q, Sun GQ, Jiang LH et al (2007) Adsorption and oxidation of ethanol on colloid-based Pt/C, PtRu/C and Pt3Sn/C catalysts: in situ FTIR spectroscopy and on-line DEMS studies. Phys Chem Chem Phys 9:2686. https://doi.org/10.1039/b700676b
Wu X, Mu F, Zhao H (2020) Recent progress in the synthesis of graphene/CNT composites and the energy-related applications. J Mater Sci Technol 55:16–34. https://doi.org/10.1016/j.jmst.2019.05.063
Dicks AL (2006) The role of carbon in fuel cells. J Power Sources 156:128–141. https://doi.org/10.1016/j.jpowsour.2006.02.054
Antolini E (2009) Carbon supports for low-temperature fuel cell catalysts. Appl Catal B 88:1–24. https://doi.org/10.1016/j.apcatb.2008.09.030
Daş E, Kaplan BY, Gürsel SA, Yurtcan AB (2019) Graphene nanoplatelets-carbon black hybrids as an efficient catalyst support for Pt nanoparticles for polymer electrolyte membrane fuel cells. Renew Energy 139:1099–1110. https://doi.org/10.1016/j.renene.2019.02.137
Kim J-P, Kim Y-K, Park C-K et al (2009) Long-term stability of various carbon-nanotube-based micro-tip emitters. Diam Relat Mater 18:486–489. https://doi.org/10.1016/j.diamond.2008.10.019
Wu G, More KL, Xu P et al (2013) A carbon-nanotube-supported graphene-rich non-precious metal oxygen reduction catalyst with enhanced performance durability. Chem Commun 49:3291. https://doi.org/10.1039/c3cc39121c
Wang R-X, Fan Y-J, Wang L et al (2015) Pt nanocatalysts on a polyindole-functionalized carbon nanotube composite with high performance for methanol electrooxidation. J Power Sources 287:341–348. https://doi.org/10.1016/j.jpowsour.2015.03.181
Zhang Y, Gu Y, Lin S et al (2011) One-step synthesis of PtPdAu ternary alloy nanoparticles on graphene with superior methanol electrooxidation activity. Electrochim Acta 56:8746–8751. https://doi.org/10.1016/j.electacta.2011.07.094
He D, Cheng K, Peng T et al (2013) Graphene/carbon nanospheres sandwich supported PEMfuel cell metal nanocatalysts with remarkably high activity and stability. J Mater Chem A 1:2126–2132. https://doi.org/10.1039/C2TA00606E
Karim NA, Shamsul NS, Alias MS, Kamarudin SK (2021) Structural and electronic properties of the adsorption molecules on Co and Fe/N-doped graphene towards the application in direct liquid fuel cell. Struct Chem 32:405–415. https://doi.org/10.1007/s11224-020-01636-3
Gao L, Yue W, Tao S, Fan L (2013) Novel strategy for preparation of graphene-Pd, Pt composite, and its enhanced electrocatalytic activity for alcohol oxidation. Langmuir 29:957–964. https://doi.org/10.1021/la303663x
Jha N, Jafri RI, Rajalakshmi N, Ramaprabhu S (2011) Graphene-multi walled carbon nanotube hybrid electrocatalyst support material for direct methanol fuel cell. Int J Hydrogen Energy 36:7284–7290. https://doi.org/10.1016/j.ijhydene.2011.03.008
Li F, Guo Y, Wu T et al (2013) Platinum nano-catalysts deposited on reduced graphene oxides for alcohol oxidation. Electrochim Acta 111:614–620. https://doi.org/10.1016/j.electacta.2013.08.058
Aqel A, El-Nour KMMA, Ammar RAA, Al-Warthan A (2012) Carbon nanotubes, science and technology part (I) structure, synthesis and characterisation. Arab J Chem 5:1–23. https://doi.org/10.1016/j.arabjc.2010.08.022
Eatemadi A, Daraee H, Karimkhanloo H et al (2014) Carbon nanotubes: Properties, synthesis, purification, and medical applications. Nanoscale Res Lett 9:393. https://doi.org/10.1186/1556-276X-9-393
Hummers WS, Offeman RE (1958) Preparation of graphitic oxide. J Am Chem Soc 80:1339–1339. https://doi.org/10.1021/ja01539a017
Antolini E (2012) Graphene as a new carbon support for low-temperature fuel cell catalysts. Appl Catal B 123–124:52–68. https://doi.org/10.1016/j.apcatb.2012.04.022
Machuno LGB, Oliveira AR, Furlan RH et al (2015) Multilayer graphene films obtained by dip coating technique. Mater Res 18:775–780. https://doi.org/10.1590/1516-1439.005415
Inada M, Enomoto N, Hojo J, Hayashi K (2017) Structural analysis and capacitive properties of carbon spheres prepared by hydrothermal carbonization. Adv Powder Technol 28:884–889. https://doi.org/10.1016/j.apt.2016.12.014
Mi Y, Hu W, Dan Y, Liu Y (2008) Synthesis of carbon micro-spheres by a glucose hydrothermal method. Mater Lett 62:1194–1196. https://doi.org/10.1016/j.matlet.2007.08.011
Saida T, Sakakibara K, Igami R, Maruyama T (2022) Synthesis of a Pt/carbon-sphere catalyst and evaluation of its oxygen reduction reaction activity in acidic environments. Energy Fuels 36:1027–1033. https://doi.org/10.1021/acs.energyfuels.1c03834
Tang K, Fu L, White RJ et al (2012) Hollow carbon nanospheres with superior rate capability for sodium-based batteries. Adv Energy Mater 2:873–877. https://doi.org/10.1002/aenm.201100691
Patil UV, Pawbake AS, Machuno LGB et al (2016) Effect of plasma treatment on multilayer graphene: X-ray photoelectron spectroscopy, surface morphology investigations and work function measurements. RSC Adv 6:48843–48850. https://doi.org/10.1039/C6RA03046G
White RJ, Tauer K, Antonietti M, Titirici M-M (2010) Functional hollow carbon nanospheres by latex templating. J Am Chem Soc 132:17360–17363. https://doi.org/10.1021/ja107697s
Bastos TL, Gelamo RV, Colmati F (2023) Carbon-graphene hybrid supporting platinum–tin electrocatalyst to enhance ethanol oxidation reaction. J Appl Electrochem. https://doi.org/10.1007/s10800-023-02027-2
Borchert H, Shevchenko EV, Robert A et al (2005) Determination of nanocrystal sizes: A comparison of TEM, SAXS, and XRD studies of highly monodisperse CoPt 3 particles. Langmuir 21:1931–1936. https://doi.org/10.1021/la0477183
Niu S, Li S, Du Y et al (2020) How to reliably report the overpotential of an electrocatalyst. ACS Energy Lett 5:1083–1087
Guo JW, Zhao TS, Prabhuram J et al (2005) Preparation and characterization of a PtRu/C nanocatalyst for direct methanol fuel cells. Electrochim Acta 51:754–763. https://doi.org/10.1016/j.electacta.2005.05.056
Désilets C, Lasia A (2012) Dynamic impedance study of ethanol and acetaldehyde oxidation at platinum in acid solutions. Electrochim Acta 78:286–293. https://doi.org/10.1016/j.electacta.2012.05.102
Zhang L, Wang L, Holt CMB et al (2012) Highly corrosion resistant platinum–niobium oxide–carbon nanotube electrodes for the oxygen reduction in PEM fuel cells. Energy Environ Sci 5:6156. https://doi.org/10.1039/c2ee02689a
Lazanas ACh, Prodromidis MI (2023) Electrochemical impedance spectroscopy─a tutorial. ACS Measurement Science Au 3:162–193. https://doi.org/10.1021/acsmeasuresciau.2c00070
Ribeiro J (2020) Electrochemical impedance spectroscopy a tool on the electrochemical investigations. Revista Virtual de Quimica 12:1626–1641. https://doi.org/10.21577/1984-6835.20200123
Irazoque S, López-Suárez A, Zagal-Padilla CK, Gamboa SA (2024) Synthesis of Pd-Cu/TPPCu electrocatalyst for direct ethanol fuel cell applications. J Appl Electrochem 54:767–781. https://doi.org/10.1007/s10800-023-02003-w
Arvay A, Yli-Rantala E, Liu C-H, Peng X-H, Koski P, Cindrella L, Kauranen P, Wilde PM, Kannan AM (2012) Characterization techniques for gas diffusion layers for proton exchange membrane fuel cells - A review. J Power Sources 213:317–337. https://doi.org/10.1016/j.jpowsour.2012.04.026
Santos DM, Paganoto GT, Queiroz MAR et al (2017) Influence of support material of PtSnNiGa C electrocatalysts for ethanol oxidation. Orbital 9:140–150. https://doi.org/10.17807/orbital.v9i3.949
Funding
The authors thank the CNPq, grants 554569/2010–8 and 475609/2008–5, and FAPEG, grant 201201270750433, for financial support. FC thanks FAPEG, grant 202110267000537. TLB thanks Capes from scholarship, 88887.701552/2022–00. Rogério V. Gelamo would like to thank the National Council Scientific and Technological Development (CNPq) (grants 440726/2020–4 and 302582/2021–5), Fapemig (Grant APQ-01359–21), and the Brazilian Institute of Science and Technology (INCT) in Carbon Nanomaterials and Nacional de Grafite Ltda.
Author information
Authors and Affiliations
Corresponding author
Additional information
Publisher's Note
Springer Nature remains neutral with regard to jurisdictional claims in published maps and institutional affiliations.
Highlights
• Graphene supporting improved Pt3Sn electrocatalysts for ethanol oxidation reaction.
• Carbon allotrope comparison as supporting modified electrocatalytic activity of Pt3Sn.
• Different carbon allotropes supporting electrocatalytic enhanced the ethanol oxidation reaction.
Supplementary Information
Below is the link to the electronic supplementary material.
Rights and permissions
Springer Nature or its licensor (e.g. a society or other partner) holds exclusive rights to this article under a publishing agreement with the author(s) or other rightsholder(s); author self-archiving of the accepted manuscript version of this article is solely governed by the terms of such publishing agreement and applicable law.
About this article
Cite this article
Bastos, T.L., Gelamo, R.V. & Colmati, F. The influence of carbon spheres and graphene synthesized by thermal method as platinum-tin electrocatalyst supports to enhance the ethanol oxidation. J Solid State Electrochem (2024). https://doi.org/10.1007/s10008-024-05980-w
Received:
Revised:
Accepted:
Published:
DOI: https://doi.org/10.1007/s10008-024-05980-w