Abstract
A CFD model based on the Reynolds Averaged Navier–Stokes (RANS) approach combined with a detailed chemical kinetic mechanism to investigate a turbulent CH4/H2/N2 jet diffusion flame is developed. The CFD governing equations of momentum, mass, and energy in the turbulent field were solved in conjunction with the standard k − ε turbulence model. The laminar flamelet concept that views the turbulent diffusion flame as an ensemble of laminar diffusion flamelets is adopted. The coupling between turbulence and chemistry is achieved by the statistical description of three parameters namely (1) mixture fraction (Z), (2) variance of mixture fraction (Z”2) and (3) scalar dissipation rate (χ). The flamelet model consists of two steps namely (a) the generation of a set of laminar flamelet solutions and (b) the integration of the laminar flamelet solutions with presumed-shape Probability Density Function (PDF). The GRI Mech-3.0 mechanism that involves 53 species and 325 reactions is adopted. The effect of various parameters such as C1ε constant in the turbulent dissipation transport equation on the numerical solution is highlighted. Also, the comparison between the CFD model results and the experimental data of velocity, temperature and mass fractions of species (CH4, H2, N2, H2O, CO2, O2 and CO) along the centreline as well as on the radial position of x/D = 5, 40 are presented. Generally, the CFD results show a good agreement with the experimental data, and the presented approach in this paper is an accurate promising alternative to LES and DNS approaches for the modelling of non-premixed turbulent configurations.












Similar content being viewed by others
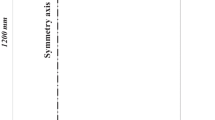
References
Williams FA (2018) Combustion theory: The fundamental theory of chemically reacting flow systems, 2nd edn. https://doi.org/10.1201/9780429494055
Masri AR, Kalt PAM, Al-Abdeli YM, Barlow RS (2007) Turbulence-chemistry interactions in non-premixed swirling flames. Combust Theory Model 11(5):653–673. https://doi.org/10.1080/13647830701213482
Zhou X, Luo KH, Williams JJR (2001) Numerical studies on vortex structures in the near-field of oscillating diffusion flames. Heat Mass Transf und Stoffuebertragung 37(2–3):101–110. https://doi.org/10.1007/s002310000166
Snegirev A, Markus E, Kuznetsov E, Harris J, Wu T (2018) On soot and radiation modeling in buoyant turbulent diffusion flames. Heat Mass Transf und Stoffuebertragung 54(8):2275–2293. https://doi.org/10.1007/s00231-017-2198-x
Yan ZH (2007) Large eddy simulations of a turbulent thermal plume. Heat Mass Transf und Stoffuebertragung 43(6):503–514. https://doi.org/10.1007/s00231-006-0127-5
Jones WP (2002) Large Eddy Simulation of turbulent combustion processes. Comput Phys Commun 147(1–2):533–537. https://doi.org/10.1016/S0010-4655(02)00330-2
Di Mare F, Jones WP, Menzies KR (2004) Large eddy simulation of a model gas turbine combustor. Combust Flame 137(3):278–294. https://doi.org/10.1016/j.combustflame.2004.01.008
Bouras F, Khaldi F (2016) Computational modeling of thermodynamic irreversibilities in turbulent non-premixed combustion. Heat Mass Transf und Stoffuebertragung 52(4):671–681. https://doi.org/10.1007/s00231-015-1587-2
Tabet F, Sarh B, Birouk M, Gökalp I (2012) The near-field region behaviour of hydrogen-air turbulent non-premixed flame. Heat Mass Transf und Stoffuebertragung 48(2):359–371. https://doi.org/10.1007/s00231-011-0889-2
Tabet F, Sarh B, Gökalp I (2011) Investigation of turbulence models capability in predicting mixing in the near field region of hydrogen-hydrocarbon turbulent non-premixed flame. Heat Mass Transf und Stoffuebertragung 47(4):397–406. https://doi.org/10.1007/s00231-010-0725-0
Li Z, Cuoci A, Sadiki A, Parente A (2017) Comprehensive numerical study of the Adelaide Jet in Hot-Coflow burner by means of RANS and detailed chemistry. Energy 139:555–570. https://doi.org/10.1016/j.energy.2017.07.132
Hafid M, Hebbir N, Lacroix M, Joly P (2022) Simulating of non ‑ premixed turbulent combustion using a presumed probability density function method. Heat Mass Transf no. 0123456789. https://doi.org/10.1007/s00231-022-03238-7
Peters N (1984) Laminar Diffusion Flametet Models in Non-Premixedturbulent Cumbustion vol. 10
Pei Y, Hawkes ER, Kook S (2013) Transported probability density function modelling of the vapour phase of an n-heptane jet at diesel engine conditions. Proc Combust Inst 34(2):3039–3047. https://doi.org/10.1016/j.proci.2012.07.033
Maghbouli A, Akkurt B, Lucchini T, D’Errico G, Deen NG, Somers B (2019) Modelling compression ignition engines by incorporation of the flamelet generated manifolds combustion closure. Combust Theory Model 23(3):414–438. https://doi.org/10.1080/13647830.2018.1537522
Pei Y, Hawkes ER, Kook S, Goldin GM, Lu T (2015) Modelling n-dodecane spray and combustion with the transported probability density function method. Combust Flame 162(5):2006–2019. https://doi.org/10.1016/j.combustflame.2014.12.019
Pope SB (1985) PDF methods for turbulent reactive flows. Prog Energy Combust Sci 11(2):119–192. https://doi.org/10.1016/0360-1285(85)90002-4
Zhang K, Ghobadian A, Nouri JM (2017) Comparative study of non-premixed and partially-premixed combustion simulations in a realistic Tay model combustor. Appl Therm Eng 110:910–920. https://doi.org/10.1016/j.applthermaleng.2016.08.223
Coclite A, Cutrone L, Gurtner M, De Palma P, Haidn OJ, Pascazio G (2016) Computing supersonic non-premixed turbulent combustion by an SMLD flamelet progress variable model. Int J Hydrogen Energy 41(1):632–646. https://doi.org/10.1016/j.ijhydene.2015.10.086
Dhuchakallaya I, Watkins AP (2009) Self-ignition of diesel spray combustion. Heat Mass Transf und Stoffuebertragung 45(12):1627–1635. https://doi.org/10.1007/s00231-009-0537-2
Ihme M, Pitsch H (2008) Prediction of extinction and reignition in nonpremixed turbulent flames using a flamelet/progress variable model. 2. Application in LES of Sandia flames D and E. Combust Flame 155(1–2):90–107. https://doi.org/10.1016/j.combustflame.2008.04.015
Van Oijen JA, De Goey LPH (2000) Combustion science and technology modelling of premixed laminar flames using flamelet-generated manifolds. Combust Sci Technol 161(1):113–137
De Goey LPH, Ten Thije Boonkkamp JHM (1999) A flamelet description of premixed laminar flames and the relation with flame stretch. Combust Flame 119(3):253–271. https://doi.org/10.1016/S0010-2180(99)00052-8
Elattar HF, Specht E, Fouda A, Bin-Mahfouz AS (2016) CFD modeling using PDF approach for investigating the flame length in rotary kilns. Heat Mass Transf und Stoffuebertragung 52(12):2635–2648. https://doi.org/10.1007/s00231-016-1768-7
Hashemi SA, Fattahi A, Sheikhzadeh GA, Mehrabian MA (2012) The effect of oxidant flow rate on a coaxial oxy-fuel flame. Heat Mass Transf und Stoffuebertragung 48(9):1615–1626. https://doi.org/10.1007/s00231-012-1008-8
Khaldi N, Chouari Y, Mhiri H, Bournot P (2016) CFD investigation on the flow and combustion in a 300 MWe tangentially fired pulverized-coal furnace. Heat Mass Transf und Stoffuebertragung 52(9):1881–1890. https://doi.org/10.1007/s00231-015-1710-4
Ben Sik Ali A, Kriaa W, Mhiri H, Bournot P (2012) Numerical investigations of cooling holes system role in the protection of the walls of a gas turbine combustion chamber. Heat Mass Transf und Stoffuebertragung 48(5):779–788. https://doi.org/10.1007/s00231-011-0932-3
Gürtürk M, Oztop HF, Pambudi NA (2018) CFD analysis of a rotary kiln using for plaster production and discussion of the effects of flue gas recirculation application. Heat Mass Transf und Stoffuebertragung 54(10):2935–2950. https://doi.org/10.1007/s00231-018-2336-0
Dally BB, Fletcher DF, Masri AR (1998) Flow and mixing fields of turbulent bluff-body jets and flames. Combust Theory Model 2(2):193–219. https://doi.org/10.1088/1364-7830/2/2/006
OpenFOAM. www.openfoam.com
Lipatnikov AN, Sabelnikov VA (2020) An extended flamelet-based presumed probability density function for predicting mean concentrations of various species in premixed turbulent flames. Int J Hydrogen Energy 45(55):31162–31178. https://doi.org/10.1016/j.ijhydene.2020.08.083
Law WP, Gimbun J (2019) Thermal performance enhancement of non-premixed syngas combustion in a partial combustion unit by winged nozzle: Experimental and CFD study. Energy 182:148–158. https://doi.org/10.1016/j.energy.2019.06.040
Okafor EC et al (2018) Experimental and numerical study of the laminar burning velocity of CH4–NH3–air premixed flames. Combust Flame 187:185–198. https://doi.org/10.1016/j.combustflame.2017.09.002
Patankar S (1980) Numerical heat transfer and fluid flow. https://doi.org/10.1016/j.watres.2009.11.010
Liu S (2016) CFD with OpenSource software Implementation of a Complete Wall Function for the Standard k − Turbulence. Proc CFD with OpenSource Softw 2016
El-Mahallawy F, Habik SE-D (2002) Fundamentals and Technology of Combustion. Elsevier Sci
Janicka J, Peters N (1982) Using a Pdf Transport Equation. Ninet Symp Combust Combust Inst, pp 367–374
Namer I, Ötügen MV (1988) Velocity measurements in a plane turbulent air jet at moderate Reynolds numbers. Exp Fluids 6(6):387–399. https://doi.org/10.1007/BF00196484
Schadow KC, Gutmark E, Koshigoe S, Wilson KJ (1989) Combustion-related shear-flow dynamics in elliptic supersonic jets. AIAA J 27(10):1347–1353. https://doi.org/10.2514/3.10270
Acknowledgements
The authors are grateful to Future Optimization Ideas Inc. for the financial support.
Author information
Authors and Affiliations
Corresponding authors
Ethics declarations
Conflict of interest
The authors declare that they have no conflicts of interest with respect to research, authorship and/or publication.
Additional information
Publisher's Note
Springer Nature remains neutral with regard to jurisdictional claims in published maps and institutional affiliations.
Highlights
• Numerical study of non-premixed flame with detailed chemistry, using OpenFOAM, is considered.
• Turbulence-chemistry interaction based on flamelet concept is adopted.
• Integration of flamelet library with presumed-PDF is performed.
• Increasement of ‘Cɛ1’ gives better prediction of the velocity.
Rights and permissions
Springer Nature or its licensor holds exclusive rights to this article under a publishing agreement with the author(s) or other rightsholder(s); author self-archiving of the accepted manuscript version of this article is solely governed by the terms of such publishing agreement and applicable law.
About this article
Cite this article
Hafid, M., Hebbir, N., Lacroix, M. et al. CFD modelling of a turbulent CH4/H2/N2 jet diffusion flame with detailed chemistry. Heat Mass Transfer 59, 583–598 (2023). https://doi.org/10.1007/s00231-022-03283-2
Received:
Accepted:
Published:
Issue Date:
DOI: https://doi.org/10.1007/s00231-022-03283-2