Abstract
Background
The study aimed to identify the targeting genes and miRNAs using the microarray expression profile dataset for Osteoarthritis (OA) patients. Differentially expressed genes (DEGs) between OA and control samples were identified using Bayes method of limma package. Subsequently, a protein-protein interaction (PPI) network was constructed. miRNAs and transcription factor (TFs) based on DEGs in PPI network were identified using Webgestalt and ENCODE, respectively. Finally, MCODE, Gene Ontology (GO) function, and Kyoto Encyclopedia of Genes and Genomes (KEGG) were performed. The expressions of several DEGs and predicted miRNAs in OA rats were detected by RT-PCR.
Results
A total of 594 DEGs were identified. In PPI network, there were 313 upregulated DEGs and 22 downregulated DEGs. Besides, the regulatory relationships included 467 upregulated interactions and 85 downregulated interactions (miR-124A → QKI and MAP 1B) between miRNA and DEGs in PPI network. The module from downregulated DEGs-TFs-miRNA networks was mainly enriched to low-density lipoprotein particle clearance, response to linoleic acid, and small molecule metabolic process BP terms. Moreover, QKI, MAP 1B mRNA and miR-9 expressions were significantly reduced in OA rats.
Conclusion
miR-9 might be a protective factor for OA patients via inhibiting proliferation and differentiation of cartilage progenitor cells. miR-124A might play an important role in progression of OA through targeting QKI and MAP 1B.
Similar content being viewed by others
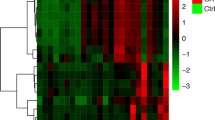
Background
Osteoarthritis (OA) is the most prevalent rheumatic disease with the characteristics of chronic, debilitating and degenerative disease of the joints [1]. Approximately 1/3 of OA patients is over 60 years old [2]. Besides, OA often occurs in people aged 60–70 years, more frequently in females (18%) than males (10%) [1] . Currently, treatments are only used to manage symptoms due to complex etiology [3]. Although some pathogenesis pathways have been reported, current study is incomplete for prevention or treatment. These limitations might be surmounted through investigating the molecular mechanisms in OA.
microRNAs (miRNAs), as novel regulators of gene expression, play key roles in biological processes of OA [4]. Previous study has found that some miRNAs associates with musculoskeletal system [5,6,7]. miR-140 is involved in the cartilage of mouse embryos during the development of long and flat bone through directly targeting distone deacetylase 4 (HDAC4) [8]. In addition, miR-140 protects against OA development by targeting ADAM metallopeptidase with thrombospondin type 1 motif 5 (Adamts-5) expression [9]. The tumor necrosis factor a (TNF-α) is an important inflammatory factor, and its expression level is increased in OA synovial membranes [10]. Decreased expression of miR-130a, correlated with TNF-α in the development of OA [6]. Thus, miR-140 and miR-130a are novel therapeutic targets in OA development. Therefore, it is necessary to identify more miRNAs associated with OA development in the future.
The present study aimed to identify the targeting genes and miRNAs using the microarray expression profile dataset. The differentially expressed genes (DEGs) were identified using Bayes method of limma package. Subsequently, a protein-protein interaction (PPI) network for these DEGs was constructed. miRNAs and transcription factor (TFs) based on DEGs in PPI network were identified using Webgestalt and ENCODE, respectively. Finally, significant network modules were obtained by MCODE, and their Gene Ontology (GO) function and Kyoto Encyclopedia of Genes and Genomes (KEGG) were enriched.
Results
The results of DEGs involved in the function and pathway for OA
Totally, 594 DEGs were identified between OA and control samples, including 535 upregulated DEGs (MMP2, FOS, et al) and 59 downregulated DEGs (QKI, MAPB1, et al). The up-regulated DEGs were enriched to 1166 BP terms, 20 KEGG pathways, and 83 Reactome pathways. The top 5 of these were showed in Table 1. The down-regulated DEGs were enriched to 480 BP terms, 4 KEGG pathways, and 27 Reactome pathways. The top 5 of these were showed in Table 2.
The DEGs-TFs-miRNA integrated network
In PPI network, there were 313 upregulated DEGs with 841 interactions and 22 downregulated DEGs with 31 interactions (Fig. 1). Among them, 25 upregulated DEGs (GLIS2, FOSB, et al) and one downregulated DEG (MYF6) were also TFs, which have a transcriptional regulatory relationship with other DEGs. In addition, the regulatory relationships between miRNA and DEGs in PPI network were also identified (e.g. miR-124A → QKI and MAP 1B), including 467 upregulated interactions and 85 downregulated interactions. The DEGs-TFs-miRNA networks based on upregulated and downregulated DEGs are showed in Figs. 2 and 3, respectively. The nodes with degree more than 20 in upregulated DEGs-TFs-miRNA network are showed in Table 3, which presents 17 miRNAs (eg., miRNA-9) and 9 TFs. In addition, in the downregulated DEGs-TFs-miRNA network, QKI had the largest degree value (degree = 21) and only one MYF6 TF was predicted. There were 4 miRNAs (eg., miR-124A) and 11 DEGs (eg., QKI and MAP 1B) in top 15 nodes listed by degree (Table 4), and QKI and MAP 1B were both predicted as target of miR-124A.
The protein-protein interaction (PPI) network for upregulated differentially expressed genes (DEGs) (a) and downregulated DEGs (b). Red nodes indicate upregulated DEGs, green nodes indicate downregulated DEGs, and the black lines indicate the interactions. The node is larger, and it degree is higher, suggesting that it has more interactions with other proteins in PPI network
The integrated network based on upregulated DEGs, transcription factor (TFs), and miRNA. Red circles indicate DEGs, green triangles indicate TFs, and blue rhombus indicate miRNAs. Arrows indicate transcriptional regulatory relationship, T-type lines indicate miRNA regulation relationship, straight lines indicate PPIs
The integrated network based on downregulated DEGs, transcription factor (TFs), and miRNA. Green circles indicate DEGs, red triangles indicate TFs, and blue rhombus indicate miRNAs. Arrows indicate transcriptional regulatory relationship, T-type lines indicate miRNA regulation relationship, straight lines indicate PPIs
The significant network modules in the integrated network
Overall, 12 modules were identified from the upregulated network. The module with the highest Mcode score (5.739) included 24 nodes and 66 interactions (Fig. 4a). These DEGs in this module were mainly enriched to extracellular matrix organization, extracellular matrix disassembly, and collagen catabolic process BP terms, as well as ECM-receptor interaction, focal adhesion, and PI3K-Akt signaling pathways. Moreover, only one module was obtained from the downregulated network (Mcode score = 6.667), including 7 nodes and 20 interactions (Fig. 4b). They were mainly enriched to low-density lipoprotein particle clearance, response to linoleic acid, and small molecule metabolic process BP terms.
The results of qRT-PCR
As shown in Fig. 5, expressions of QKI Mrna (P < 0.01) and miR-9 (P < 0.05) were significantly reduced in OA rats. Although expression of MAP 1B was also reduced in synovial tissue of OA rats, there were no significant differences when compared with that of health rats.
Discussion
In this study, 535 upregulated DEGs and 59 downregulated DEGs (e.g. QKI and MAPB1) were identified between OA and control samples. Among them, 313 upregulated DEGs with 841 interactions and 22 downregulated DEGs with 31 interactions were used to construct PPI network. As a result, 25 upregulated DEGs and one downregulated DEG in PPI network having a transcriptional regulatory relationship with other DEGs were obtained. In upregulated DEGs-TFs-miRNA network, miR-9 had higher degree values and was predicted to regulate 22 targets. Besides, the regulatory relationships (e.g. miR-124A → QKI and MAP 1B) were highlighted in downregulated DEGs-TFs-miRNA network, in which QKI had the largest degree value, and QKI and MAP 1B in top 15 nodes were both predicted as target of miR-124A. The module from downregulated DEGs-TFs-miRNA networks was mainly enriched to low-density lipoprotein particle clearance, response to linoleic acid, and small molecule metabolic process BP terms. Moreover, it was confirmed that expressions of QKI, MAP 1B mRNA and miR-9 were significantly reduced in OA rats.
As is described above, miRNAs are novel regulators of gene expression and play key roles in biological processes of OA. miR-9 expression is upregulated in osteosarcoma tissues when compared with human osteoblastic hFOB cells and the corresponding non-cancerous bone tissues [11]. Whereas osteosarcoma is derived from progenitor cells, which can be differentiated into bone, cartilage and fiber [12]. In other word, cartilage contain a number of stem cells or progenitor cells, which has been confirmed by a previous study [13]. In addition, Jiang et al. have found that cartilage-derived stem/progenitor cells might have a key role in cartilage repair, which will behave therapeutic potential in OA [14]. MiR-9 has been identified to play critical roles in proliferation and differentiation of retinal progenitor cell and neural progenitor cells [15, 16]. In a round-about way, miR-9 might play a consequence role in OA through regulating proliferation and differentiation of cartilage progenitor cell for cartilage repair. In the present study, miR-9 expression is reduced in OA rats when compared with the healthy rats, suggesting miR-9 is involved in pathological process of OA. Similarly, a previous study indicates that expression level of miR-9 is decreased in OA chondrocytes with numerous apoptotic cell deaths through upregulating PRTG [17]. Therefore, miR-9 might be a novel protective factor for OA patients via inhibiting proliferation and differentiation of cartilage progenitor cells.
In the present study, KH Domain Containing RNA Binding (QKI) and Microtubule Associated Protein 1B (MAP 1B) were predicted as target genes of miR-124A. miR-124 plays roles in various pathologic conditions such as inflammatory responses, and osteoclast differentiation [18] miR-124A is overexpressed in OA cartilage with cocultured chondrocytes derived from MSC [19, 20]. Notably, it shows the alterations in chondrogenesis and neurogenesis are associated with the development of OA [21]. QKI belongs to a family of RNA-binding proteins called STAR proteins for Signal Transduction and Activation of RNA [22]. Recently, a study has confirmed that QKI genes is significantly suppressed when synovial explants were treated with miR-27b-3p in OA patients [23]. MAP 1B belongs to the microtubule-associated protein family and is involved in microtubule assembly [24]. Actin cytoskeleton and microtubule array play essential roles on chondrogenesis [25]. It has been reported that expression of MAP 1B in mRNA and protein levels is significantly upregulated in degraded cartilage by Steinberg [26]. The 3′UTR of MAP 1B mRNA interacts with QKI resulted in reducing MAP 1B mRNA expression in the QKI-deficiency mutant mice. Meanwhile, RNAi-mediated QKI-knockdown caused destabilization of the MAP 1B mRNA in oligodendroglia CG4 cell line [27]. Furthermore, overexpression of exogenous QKI was able to facilitate MAP 1B expression. Interesting, QKI and MAP 1B mRNA expression levels were also reduced in rats with OA in this study. Therefore, we inferred miR-124A might play an important role in progression of OA through targeting QKI and MAP 1B.
However, there are still some limitations in the study. All of miRNAs in DEGs-TFs-miRNAs were predicted in OA samples using bioinformatic analysis, and their expressions and regulatory relationships should be verified using future experiments. In addition, these results will be confirmed using human samples. Moreover, the expression of MAP 1B was reduced in synovial tissue of OA rats than in health rats, but no significant differences, which should be verified in large samples in future.
Conclusion
miR-9 might be a novel protective factor for OA patients OA synovia via inhibiting proliferation and differentiation of cartilage progenitor cells. In addition, miR-124A might play an important role in progression of OA through targeting QKI and MAP 1B. This study will provide important clues for understanding of the mechanisms of OA and the development of therapeutic targets for OA.
Methods
Data acquisition and preprocessing
The expression profile dataset GSE82107 was downloaded from the Gene Expression Omnibus (GEO; http://www.ncbi.nlm.nih.gov/geo/) database. The data was deposited into the following platform: GPL570 [HG-U133_Plus_2] (Affymetrix Human Genome U133 Plus 2.0 Array) by Marieke et al., including 10 end-stage OA synovial biopsies from the Orthopedics department of the Radboud University Medical Center and 7 synovial biopsies from individuals without a joint disease [28]. The study protocols were approved by CMO region Arnhem-Nijmegen in Netherlands.
Identification of DEGs
The data was normalized using RMA function of Affy package in R software (http://www.bioconductor.org/packages/release/bioc/html/affy.html). Subsequently, DEGs between OA and control samples were identified using Bayes method of limma package in R software (http://www.bioconductor.org/packages/release/bioc/html/limma.html). The threshold value of DEGs was set as |log fold change (FC)| > 1.0 and P value < 0.05.
GO enrichment function and pathway analyses
The GO function, KEGG pathway and Reactome pathway enrichment analyses were performed to reveal the DEGs involved in biological processes (BP) and pathways through an online database (www.biocloudservice.com). P value < 0.05 was used as the significant difference.
Construction of the PPI network
Proteins rarely act alone as their functions tend to be regulated. However, PPI network can provide a valuable framework to understand the functional organization of the proteome [29]. Therefore, the PPIs of DEGs was analyzed using STRING online tool (version 10.0). DEGs with combined score > 0.4 were identified.
Identification of miRNAs and TFs based on DEGs in PPI network
Webgestalt was used to identify miRNAs that regulated DEGs in PPI network under the threshold value of gene count ≥4 and adjusted P values < 0.05. In addition, TFs were screened from DEGs in the PPI network using ENCODE database [30].
The integrated network using DEGs in the PPI network, miRNA, and TFs
DEGs in PPI network and regulation relationships of miRNA-DEGs and TFs-DEGs were integrated to a network using Cytoscape (version 3.2.0, http://www.cytoscape.org/). As we known, the integrated network subjected to scale-free network. So the hub proteins were identified using connectivity degree analysis.
Module analysis based on the integrated network
MCODE was used to generate a sub-network for a list of significant network modules. The default threshold was set as following: Degree Cutoff: 2, Node Score Cutoff: 0.2, K-Core: 2, Max. Depth: 100. Subsequently, the GO function and KEGG pathway of significant network modules were enriched.
Animal model of OA
The male sprague dawley (SD) rats (weight 220 ± 25 g and 8 week-old) purchased from SLAC Laboratory Animal Ltd. Co (Shanghai, China) were used to establish the animal model of OA. All procedures were conformed to the Institutional Animal Care and approved by Committee of Laboratory Animal Center of Nantong University. Rats were randomly divided into the control and model groups (30 rats each group). After acclimatization at the third day, rats in control group were given normal food without any other treatment. The left knee joints of rats in the model group were subjected to anterior cruciate ligament transaction (ACLT) according to the previous study [31], while the right knee joints of rats were served as sham control. Briefly, rats were anesthetized with pentobarbital sodium (30 mg/kg), then shaved and disinfected to expose knee joint. The patella was dislocated laterally and knee joint was buckled as much as possible. After anterior cruciate ligament (ACL) exposed, the ACL was transected with micro-scissors. Next, the joint surface was washed with sterile saline solution, and capsule and skin were sutured. In sham group, the operations were same except for ACLT. Penicillin was injected in a dose of 400,000 units per day for three consecutive days after surgery. Subsequently, rats were sacrificed and their joints were harvested at 8 weeks post-surgery.
The verification of qRT-PCR
To confirm the above results, expression levels of QKI, MAP 1B and miR-9 in OA samples (left knee joints) and sham control (right knee joints) were detected using qRT-PCR. Total RNA was extracted from synovial tissues of rats using RNAiso Plus reagent following the manufacturer’s instructions (9109, TAKARA, Japan) under low temperature. Subsequently, the first strand cDNA was prepared from synovial tissues RNA using PrimeScript™RT Master Mix according to the manufacturer’s instructions (RR036A, TAKARA). Subsequently, the rat glyceraldehydes-3-phosphate dehydrogenase (GAPDH) was used as an internal reference. The primers used for QKI (Forward primer: AGTACCCCATTGAACCCAGC; Reverse primer: TGTCTGGTAAAACAGTGGGGT) MAP 1B (Forward primer: ACGGTAGGGATTACAACG; Reverse primer: GACTCAGGGATGGACTCTT) and GAPDH (Forward primer: AGACAGCCGCATCTTCTTGT; Reverse primer: CTTGCCGTGGGTAGAGTCAT) were designed on rat sequences. The relative amounts of mRNAs were analyzed using Relative Expression Software Tool (REST). In addition, miR-9 (rno-miR-9a-5p-RT: GTCGTATCCAGTGCAGGGTCCGAGGTATTCGCACTGGATACGACTCATAC; JH-rno-miR-9a-5p-Rf: GCGCGCTCTTTGGTTATCTAGCT) was also detected using qRT-PCR. The rat U6 (forward primer: GCTTCGGCAGCACATATACTAAAAT, reverse primer: CGCTTCACGAATTTGCGTGTCAT) was used as an endogenous control. Relative gene expression was assessed by 2−ΔΔCt method.
Statistical analysis
The gene expression values in the OA and control groups were presented as mean ± standard deviation (X ± SD), and compared using unpaired Student’s t-test by SPSS 22.0 (SPSS Inc., Chicago, IL, USA). P < 0.05 was defined to be significantly different.
Availability of data and materials
The datasets used and analysed during the current study are available from the corresponding author on reasonable request.
Abbreviations
- DEGs:
-
Differentially expressed genes
- PPI:
-
Protein-protein interaction
- TFs:
-
Transcription factor
- GO:
-
Gene Ontology
- KEGG:
-
Kyoto Encyclopedia of Genes and Genomes
- OA:
-
Osteoarthritis
- miRNAs:
-
MicroRNAs
- TNF-α:
-
Tumor necrosis factor a
- GEO:
-
Gene Expression Omnibus
- BP:
-
Biological processes
References
Glynjones S, Palmer AJ, Agricola R, Price AJ, Vincent TL, Weinans H, et al. Osteoarthritis. Lancet. 2015;386(9991):376–87.
Collaborators IP. Global, regional, and national incidence, prevalence, and years lived with disability for 310 diseases and injuries, 1990-2015: a systematic analysis for the global burden of disease study 2015. Lancet. 2016;388(10053):1545–602.
Harris KP, Driban JB, Sitler MR, Cattano NM, Balasubramanian E, Hootman JM. Tibiofemoral osteoarthritis after surgical or nonsurgical treatment of anterior cruciate ligament rupture: a systematic review. J Athl Train. 2017;52(6):507–17.
Miyaki S, Asahara H. Macro view of microRNA function in osteoarthritis. Nat Rev Rheumatol. 2012;8(9):543–52.
Murata K, Yoshitomi H, Tanida S, Ishikawa M, Nishitani K, Ito H, et al. Plasma and synovial fluid microRNAs as potential biomarkers of rheumatoid arthritis and osteoarthritis. Arthritis Res Ther. 2010;12(3):R86.
Li Z-C, Han N, Li X, Li G, Liu Y-Z, Sun G-X, et al. Decreased expression of microRNA-130a correlates with TNF-α in the development of osteoarthritis. Int J Clin Exp Pathol. 2015;8(3):2555.
Kobayashi T, Lu J, Cobb BS, Rodda SJ, McMahon AP, Schipani E, et al. Dicer-dependent pathways regulate chondrocyte proliferation and differentiation. Proc Natl Acad Sci. 2008;105(6):1949–54.
Tuddenham L, Wheeler G, Ntounia-Fousara S, Waters J, Hajihosseini MK, Clark I, et al. The cartilage specific microRNA-140 targets histone deacetylase 4 in mouse cells. FEBS Lett. 2006;580(17):4214–7.
Miyaki S, Sato T, Inoue A, Otsuki S, Ito Y, Yokoyama S, et al. MicroRNA-140 plays dual roles in both cartilage development and homeostasis. Genes Dev. 2010;24(11):1173–85.
Fernandes JC, Martel-Pelletier J, Pelletier JP. The role of cytokines in osteoarthritis pathophysiology. Biorheology. 2002;39(1, 2):237–46.
Qi XJ, Wang JF, Wang GD, Xu Q, Sun HL. Pivotal role of microRNA-9 in osteosarcoma tumorigenesis and tumor progression. Genet Mol Res Gmr. 2016;15(1):gmr.15017318. https://doi.org/10.4238/gmr.15017318.
Jiang Y, Cai Y, Zhang W, Yin Z, Hu C, Tong T, et al. Human cartilage-derived progenitor cells from committed chondrocytes for efficient cartilage repair and regeneration. Stem Cells Transl Med. 2016;5(6):733–44.
Hayes AJ, Tudor D, Nowell MA, Caterson B, Hughes CE. Chondroitin sulfate Sulfation motifs as putative biomarkers for isolation of articular cartilage progenitor cells. J Histochem Cytochem Off J Histochem Soc. 2008;56(2):125.
Jiang Y, Tuan RS, et al. Origin and function of cartilage stem/progenitor cells in osteoarthritis Nat Rev Rheumatol. 2015;11(4):206–12.
Topol A, Zhu S, Hartley BJ, English J, Hauberg ME, Readhead B, et al. Dysregulation of miRNA-9 in a subset of schizophrenia patient-derived neural progenitor cells. Cell Rep. 2016;15(5):1024.
Hu Y, Luo M, Ni N, Den Y, **a J, Chen J, et al. Reciprocal actions of microRNA-9 and TLX in the proliferation and differentiation of retinal progenitor cells. Stem Cells Dev. 2014;23(22):2771–81.
Song J, Kim D, Chun CH, ** EJ. MicroRNA-9 regulates survival of chondroblasts and cartilage integrity by targeting protogenin. Cell Communication Signaling. 2013;11(1):66.
Nakamachi Y, Saegusa J, Kawano S. MicroRNA-124: a promising therapeutic agent for various human diseases, including rheumatoid arthritis. RNA DIS. 2017;4:76047028. https://doi.org/10.14800/rd.1252.
Miranda-Duarte A. Epigenetic mechanisms in osteoarthritis. In: Qian C, editor. Osteoarthritis: Progress Basic Res Treatment, IntechOpen. 2015. p. 15–38.
Mayer U, Springorum H-R, Grässel S. Influence of osteoarthritic cartilage explants on the expression of MIRNAS and predicted targets in cocultured chondrocytes and mesenchymal stem cells. Osteoarthr Cartil. 2016;24:S346.
Yu X-M, Meng H-Y, Yuan X-L, Wang Y, Guo Q-Y, Peng J, et al. MicroRNAs’ involvement in osteoarthritis and the prospects for treatments. Evid Based Complement Alternat Med. 2015;2015:1–13.
Vernet C, Artzt K. STAR, a gene family involved in signal transduction and activation of RNA. Trends Genet. 1997;13(12):479.
Li YH, Tavallaee G, Tokar T, Nakamura A, Sundararajan K, Weston A, et al. Identification of synovial fluid microRNA signature in knee osteoarthritis: differentiating early- and late-stage knee osteoarthritis. Osteoarthritis Cartilage. 2016;24(9):1577.
Gevorkian G, Gonzaleznoriega A, Acero G, Ordoñez J, Michalak C, Munguia ME, et al. Amyloid-beta peptide binds to microtubule-associated protein 1B (MAP 1B). Neurochem Int. 2008;52(6):1030–6.
Tvorogova AA, Kovaleva AV, Saidova AA. Reorganization of actin cytoskeleton and microtubule array during the chondrogenesis of bovine MSCs. Annual Res Rev Biol. 2018;29(6):1–14.
Steinberg J, Grs R, Roumeliotis TI, Jayasuriya RL, Clark MJ, Brooks RA, et al. Integrative epigenomics, transcriptomics and proteomics of patient chondrocytes reveal genes and pathways involved in osteoarthritis. Sci Rep. 2017;7(1):8935.
Zhao L, Ku L, Chen Y, **a M, LoPresti P, Feng Y. QKI binds MAP 1B mRNA and enhances MAP 1B expression during oligodendrocyte development. Mol Biol Cell. 2006;17(10):4179–86.
Broeren MGA, Vries MD, Bennink MB, Lent PLEMV, Kraan PMVD, Koenders MI, et al. Functional tissue analysis reveals successful cryopreservation of human osteoarthritic Synovium. PLoS One. 2016;11(11):e0167076.
De LRJ, Fontanillo C. Protein-protein interactions essentials: key concepts to building and analyzing interactome networks. PLoS Comput Biol. 2010;6(6):e1000807.
Consortium EP. Identification and analysis of functional elements in 1% of the human genome by the ENCODE pilot project. Nature. 2007;447(7146):799.
Hayami T, Pickarski M, Zhuo Y, Wesolowski GA, Rodan GA, Duong LT. Characterization of articular cartilage and subchondral bone changes in the rat anterior cruciate ligament transection and meniscectomized models of osteoarthritis. Bone. 2006;38(2):234–43.
Acknowledgements
None.
Funding
None.
Author information
Authors and Affiliations
Contributions
Conception and design of the research: LJ; acquisition of data: LJ, XS; analysis and interpretation of data: XS; statistical analysis: HK; drafting the manuscript: LJ; revision of manuscript for important intellectual content: HK. All authors read and approved the final manuscript.
Corresponding author
Ethics declarations
Ethics approval and consent to participate
Not applicable.
Consent for publication
Not applicable.
Competing interests
The authors declare that they have no competing interests.
Additional information
Publisher’s Note
Springer Nature remains neutral with regard to jurisdictional claims in published maps and institutional affiliations.
Rights and permissions
Open Access This article is licensed under a Creative Commons Attribution 4.0 International License, which permits use, sharing, adaptation, distribution and reproduction in any medium or format, as long as you give appropriate credit to the original author(s) and the source, provide a link to the Creative Commons licence, and indicate if changes were made. The images or other third party material in this article are included in the article's Creative Commons licence, unless indicated otherwise in a credit line to the material. If material is not included in the article's Creative Commons licence and your intended use is not permitted by statutory regulation or exceeds the permitted use, you will need to obtain permission directly from the copyright holder. To view a copy of this licence, visit http://creativecommons.org/licenses/by/4.0/. The Creative Commons Public Domain Dedication waiver (http://creativecommons.org/publicdomain/zero/1.0/) applies to the data made available in this article, unless otherwise stated in a credit line to the data.
About this article
Cite this article
Jiang, L., Sun, X. & Kong, H. microRNA-9 might be a novel protective factor for osteoarthritis patients. Hereditas 157, 15 (2020). https://doi.org/10.1186/s41065-020-00128-y
Received:
Accepted:
Published:
DOI: https://doi.org/10.1186/s41065-020-00128-y