Abstract
Porous titanium (Ti) scaffolds have been extensively utilized as bone substitute scaffolds due to their superior biocompatibility and excellent mechanical properties. However, naturally formed TiO2 on the surface limits fast osseointegration. Different biomolecules have been widely utilized to overcome this issue; however, homogeneous porous Ti scaffolds could not simultaneously deliver multiple biomolecules that have different release behaviors. In this study, functionally graded porous Ti scaffolds (FGPTs) with dense inner and porous outer parts were fabricated using a two-body combination and densification procedure. FGPTs with growth factor (BMP-2) and antibiotics (TCH) exhibited suitable mechanical properties as bone substituting material and presented good structural stability. The release of BMP-2 was considerably prolonged, whereas the release of TCH was comparable to that of homogenous porous titanium scaffolds (control group). The osteogenic differentiation obtained using FGPTs was maintained due to the prolonged release of BMP-2. The antimicrobial properties of these scaffolds were verified using S. aureus in terms of prior release time. In addition, various candidates for graded porous Ti scaffolds with altered pore characteristics were presented.
Graphic abstract









Similar content being viewed by others
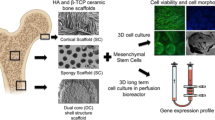
References
Cheon KH, Park C, Kang MH et al (2021) Construction of tantalum/poly (ether imide) coatings on magnesium implants with both corrosion protection and osseointegration properties. Bioact Mater 6(4):1189–1200. https://doi.org/10.1016/j.bioactmat.2020.10.007
Jang TS, Kim DE, Han G et al (2020) Powder based additive manufacturing for biomedical application of titanium and its alloys: a review. Biomed Eng Lett 10:505–516. https://doi.org/10.1007/s13534-020-00177-2
Yuan L, Ding S, Wen C (2019) Additive manufacturing technology for porous metal implant applications and triple minimal surface structures: A review. Bioact Mater 4:56–70. https://doi.org/10.1016/j.bioactmat.2018.12.003
Kaur M, Singh K (2019) Review on titanium and titanium based alloys as biomaterials for orthopaedic applications. Mater Sci Eng C 102:844–862. https://doi.org/10.1016/j.msec.2019.04.064
Lee MK, Lee H, Park C et al (2022) Accelerated biodegradation of iron-based implants via tantalum-implanted surface nanostructures. Bioact Mater 9:239–250. https://doi.org/10.1016/j.bioactmat.2021.07.003
Yook SW, Jung HD, Park CH et al (2012) Reverse freeze casting: a new method for fabricating highly porous titanium scaffolds with aligned large pores. Acta Biomater 8(6):2401–2410. https://doi.org/10.1016/j.actbio.2012.03.020
Kim SW, Jung HD, Kang MH et al (2013) Fabrication of porous titanium scaffold with controlled porous structure and net-shape using magnesium as spacer. Mater Sci Eng C 33(5):2808–2815. https://doi.org/10.1016/j.msec.2013.03.011
Jang TS, Jung HD, Kim S et al (2017) Multiscale porous titanium surfaces via a two-step etching process for improved mechanical and biological performance. Biomed Mater 12(2):025008. https://doi.org/10.1088/1748-605X/aa5d74
Lee H, Jang TS, Song J et al (2016) Multi-scale porous Ti6Al4V scaffolds with enhanced strength and biocompatibility formed via dynamic freeze-casting coupled with micro-arc oxidation. Mater Lett 185:21–24. https://doi.org/10.1016/j.matlet.2016.08.075
Chouirfa H, Bouloussa H, Migonney V et al (2019) Review of titanium surface modification techniques and coatings for antibacterial applications. Acta Biomater 83:37–54. https://doi.org/10.1016/j.actbio.2018.10.036
Sarraf M, Rezvani Ghomi E, Alipour S et al (2021) A state-of-the-art review of the fabrication and characteristics of titanium and its alloys for biomedical applications. Bio-Des Manuf. https://doi.org/10.1007/s42242-021-00170-3
Spriano S, Yamaguchi S, Baino F et al (2018) A critical review of multifunctional titanium surfaces: new frontiers for improving osseointegration and host response, avoiding bacteria contamination. Acta Biomater 79:1–22. https://doi.org/10.1016/j.actbio.2018.08.013
Huang J, Zhang X, Yan W et al (2017) Nanotubular topography enhances the bioactivity of titanium implants. Nanomed: Nanotechnol Biol Med 13(6):1913–1923. https://doi.org/10.1016/j.nano.2017.03.017
Kim J, Lee H, Jang TS et al (2021) Characterization of titanium surface modification strategies for osseointegration enhancement. Metals 11(4):618. https://doi.org/10.3390/met11040618
Lee MK, Lee H, Kim HE et al (2021) Nano-topographical control of Ti-Nb-Zr alloy surfaces for enhanced osteoblastic response. Nanomaterials 11(6):1507. https://doi.org/10.3390/nano11061507
Yin C, Zhang T, Wei Q et al (2022) Surface treatment of 3D printed porous Ti6Al4V implants by ultraviolet photofunctionalization for improved osseointegration. Bioact Mater 7:26–38. https://doi.org/10.1016/j.bioactmat.2021.05.043
He Y, Li Z, Ding X et al (2022) Nanoporous titanium implant surface promotes osteogenesis by suppressing osteoclastogenesis via integrin β1/FAKpY397/MAPK pathway. Bioact Mater 8:109–123. https://doi.org/10.1016/j.bioactmat.2021.06.033
Kuttappan S, Mathew D, Ji Jo et al (2018) Dual release of growth factor from nanocomposite fibrous scaffold promotes vascularisation and bone regeneration in rat critical sized calvarial defect. Acta Biomater 78:36–47. https://doi.org/10.1016/j.actbio.2018.07.050
Lee GH, Makkar P, Paul K et al (2017) Development of BMP-2 immobilized polydopamine mediated multichannelled biphasic calcium phosphate granules for improved bone regeneration. Mater Lett 208:122–125. https://doi.org/10.1016/j.matlet.2017.05.017
Maher S, Kaur G, Lima-Marques L et al (2017) Engineering of micro-to nanostructured 3d-printed drug-releasing titanium implants for enhanced osseointegration and localized delivery of anticancer drugs. ACS Appl Mater Interfaces 9(35):29562–29570. https://doi.org/10.1021/acsami.7b09916
Sarkar N, Bose S (2020) Controlled delivery of curcumin and vitamin K2 from hydroxyapatite-coated titanium implant for enhanced in vitro chemoprevention, osteogenesis, and in vivo osseointegration. ACS Appl Mater Interfaces 12(12):13644–13656. https://doi.org/10.1021/acsami.9b22474
Wang C, Lai J, Li K et al (2021) Cryogenic 3D printing of dual-delivery scaffolds for improved bone regeneration with enhanced vascularization. Bioact Mater 6(1):137–145. https://doi.org/10.1016/j.bioactmat.2020.07.007
Bai J, Wang H, Chen H et al (2020) Biomimetic osteogenic peptide with mussel adhesion and osteoimmunomodulatory functions to ameliorate interfacial osseointegration under chronic inflammation. Biomaterials 255:120197. https://doi.org/10.1016/j.biomaterials.2020.120197
Qureshi AT, Doyle A, Chen C et al (2015) Photoactivated miR-148b–nanoparticle conjugates improve closure of critical size mouse calvarial defects. Acta Biomater 12:166–173. https://doi.org/10.1016/j.actbio.2014.10.010
Hu B, Li Y, Wang M et al (2018) Functional reconstruction of critical-sized load-bearing bone defects using a Sclerostin-targeting miR-210-3p-based construct to enhance osteogenic activity. Acta Biomater 76:275–282. https://doi.org/10.1016/j.actbio.2018.06.017
**ng H, Wang X, **ao G et al (2020) Hierarchical assembly of nanostructured coating for siRNA-based dual therapy of bone regeneration and revascularization. Biomaterials 235:119784. https://doi.org/10.1016/j.biomaterials.2020.119784
Ständert V, Borcherding K, Bormann N et al (2021) Antibiotic-loaded amphora-shaped pores on a titanium implant surface enhance osteointegration and prevent infections. Bioact Mater 6(8):2331–2345. https://doi.org/10.1016/j.bioactmat.2021.01.012
Lorenzetti M, Dogša I, Stošicki T et al (2015) The influence of surface modification on bacterial adhesion to titanium-based substrates. ACS Appl Mater Interfaces 7(3):1644–1651. https://doi.org/10.1021/am507148n
Lee IK, Han IH, Hwang SW et al (2012) Analysis of attachment, proliferation and differentiation response of human mesenchymal stem cell to various implant surfaces coated with rhBMP-2. J Korean Acad Prosthodont 50(1):44–52. https://doi.org/10.4047/jkap.2012.50.1.44
Teng F-Y, Tai IC, Ho M-L et al (2019) Controlled release of BMP-2 from titanium with electrodeposition modification enhancing critical size bone formation. Mater Sci Eng C 105:109879. https://doi.org/10.1016/j.msec.2019.109879
Tang W, Yu Y, Wang J et al (2020) Enhancement and orchestration of osteogenesis and angiogenesis by a dual-modular design of growth factors delivery scaffolds and 26SCS decoration. Biomaterials 232:119645. https://doi.org/10.1016/j.biomaterials.2019.119645
Bai L, Chen P, Zhao Y et al (2021) A micro/nano-biomimetic coating on titanium orchestrates osteo/angio-genesis and osteoimmunomodulation for advanced osseointegration. Biomaterials 278:121162. https://doi.org/10.1016/j.biomaterials.2021.121162
Long M, Rack HJ (1998) Titanium alloys in total joint replacement–a materials science perspective. Biomaterials 19(18):1621–1639. https://doi.org/10.1016/s0142-9612(97)00146-4
Sumner DR (2015) Long-term implant fixation and stress-shielding in total hip replacement. J Biomech 48(5):797–800. https://doi.org/10.1016/j.jbiomech.2014.12.021
Abbasian Najafabadi T, Illarionov A, Belikov S et al (2017) Metallic materials for medical use. MATEC Web Conf 132:03003. https://doi.org/10.1051/matecconf/201713203003
Niinomi M, Nakai M (2011) Titanium-based biomaterials for preventing stress shielding between implant devices and bone. Int J Biomater 2011:836587. https://doi.org/10.1155/2011/836587
Vijayavenkataraman S, Gopinath A, Lu WF (2020) A new design of 3D-printed orthopedic bone plates with auxetic structures to mitigate stress shielding and improve intra-operative bending. Bio-Des Manuf 3(2):98–108. https://doi.org/10.1007/s42242-020-00066-8
Jung HD, Yook SW, Han CM et al (2014) Highly aligned porous Ti scaffold coated with bone morphogenetic protein-loaded silica/chitosan hybrid for enhanced bone regeneration. J Biomed Mater Res Part B 102(5):913–921. https://doi.org/10.1002/jbm.b.33072
Bari K, Arjunan A (2019) Extra low interstitial titanium based fully porous morphological bone scaffolds manufactured using selective laser melting. J Mech Behav Biomed Mater 95:1–12. https://doi.org/10.1016/j.jmbbm.2019.03.025
Caparros C, Ortiz-Hernandez M, Molmeneu M et al (2016) Bioactive macroporous titanium implants highly interconnected. J Mater Sci-Mater Med 27(10):151. https://doi.org/10.1007/s10856-016-5764-8
Wang C, Chen H, Zhu X et al (2017) An improved polymeric sponge replication method for biomedical porous titanium scaffolds. Mater Sci Eng C 70(Pt 2):1192–1199. https://doi.org/10.1016/j.msec.2016.03.037
Lee JB, Ahn MK, Koh YH et al (2016) Ti scaffolds with tailored porosities and mechanical properties using porous polymer templates. Mater Des 101:323–331. https://doi.org/10.1016/j.matdes.2016.03.142
Taniguchi N, Fujibayashi S, Takemoto M et al (2016) Effect of pore size on bone ingrowth into porous titanium implants fabricated by additive manufacturing: an in vivo experiment. Mater Sci Eng C 59:690–701. https://doi.org/10.1016/j.msec.2015.10.069
Jonitz-Heincke A, Wieding J, Schulze C et al (2013) Comparative analysis of the oxygen supply and viability of human osteoblasts in three-dimensional titanium scaffolds produced by laser-beam or electron-beam melting. Materials 6(11):5398–5409. https://doi.org/10.3390/ma6115398
Schouman T, Schmitt M, Adam C et al (2016) Influence of the overall stiffness of a load-bearing porous titanium implant on bone ingrowth in critical-size mandibular bone defects in sheep. J Mech Behav Biomed Mater 59:484–496. https://doi.org/10.1016/j.jmbbm.2016.02.036
Lou S, Pagani L, Zeng W et al (2019) Surface texture evaluation of additively manufactured metallic cellular scaffolds for acetabular implants using X-ray computed tomography. Bio-Des Manuf 2(2):55–64. https://doi.org/10.1007/s42242-019-00042-x
Vilardell AM, Cinca N, Garcia-Giralt N et al (2018) In-vitro study of hierarchical structures: anodic oxidation and alkaline treatments onto highly rough titanium cold gas spray coatings for biomedical applications. Mater Sci Eng C 91:589–596. https://doi.org/10.1016/j.msec.2018.05.071
Jung HD, Jang TS, Wang L et al (2015) Novel strategy for mechanically tunable and bioactive metal implants. Biomaterials 37:49–61. https://doi.org/10.1016/j.biomaterials.2014.10.027
Jung HD, Lee H, Kim HE et al (2015) Fabrication of mechanically tunable and bioactive metal scaffolds for biomedical applications. J Vis Exp 106:e53279. https://doi.org/10.3791/53279
Dong XN, Acuna RL, Luo Q et al (2012) Orientation dependence of progressive post-yield behaviour of human cortical bone in compression. J Biomech 45(16):2829–2834. https://doi.org/10.1016/j.jbiomech.2012.08.034
Wenzel RP (2010) Minimizing surgical-site infections. N Engl J Med 362(1):75–77. https://doi.org/10.1056/NEJMe0908753
Wenke JC, Guelcher SA (2011) Dual delivery of an antibiotic and a growth factor addresses both the microbiological and biological challenges of contaminated bone fractures. Expert Opin Drug Deliv 8(12):1555–1569. https://doi.org/10.1517/17425247.2011.628655
Radda’a NS, Goldmann WH, Detsch R et al (2017) Electrophoretic deposition of tetracycline hydrochloride loaded halloysite nanotubes chitosan/bioactive glass composite coatings for orthopedic implants. Surf Coat Tech 327:146–157. https://doi.org/10.1016/j.surfcoat.2017.07.048
Lee H, Lee MK, Cheon KH et al (2021) Functionally assembled metal platform as lego-like module system for enhanced mechanical tunability and biomolecules delivery. Mater Des 207:109840. https://doi.org/10.1016/j.matdes.2021.109840
Thenevin I, Blanco-Martín L, Hadj-Hassen F et al (2017) Laboratory pull-out tests on fully grouted rock bolts and cable bolts: Results and lessons learned. J Rock Mech Geotech Eng 9(5):843–855. https://doi.org/10.1016/j.jrmge.2017.04.005
Min J, Braatz RD, Hammond PT (2014) Tunable staged release of therapeutics from layer-by-layer coatings with clay interlayer barrier. Biomaterials 35(8):2507–2517. https://doi.org/10.1016/j.biomaterials.2013.12.009
Chung RJ, Ou KL, Tseng WK et al (2016) Controlled release of BMP-2 by chitosan/γ-PGA polyelectrolyte multilayers coating on titanium alloy promotes osteogenic differentiation in rat bone-marrow mesenchymal stem cells. Surf Coat Tech 303:283–288. https://doi.org/10.1016/j.surfcoat.2016.03.081
Barfeie A, Wilson J, Rees J (2015) Implant surface characteristics and their effect on osseointegration. Br Dent J 218(5):E9. https://doi.org/10.1038/sj.bdj.2015.171
Jung HD, Yook SW, Jang TS et al (2013) Dynamic freeze casting for the production of porous titanium (Ti) scaffolds. Mater Sci Eng C 33(1):59–63. https://doi.org/10.1016/j.msec.2012.08.004
Bobbert FSL, Lietaert K, Eftekhari AA et al (2017) Additively manufactured metallic porous biomaterials based on minimal surfaces: a unique combination of topological, mechanical, and mass transport properties. Acta Biomater 53:572–584. https://doi.org/10.1016/j.actbio.2017.02.024
Li Y, Jahr H, Lietaert K et al (2018) Additively manufactured biodegradable porous iron. Acta Biomater 77:380–393. https://doi.org/10.1016/j.actbio.2018.07.011
Acknowledgements
This work was supported by the National Research Foundation of Korea (NRF) grant funded by the Korea government (MSIT) (Nos. 2021R1I1A1A01043176 and 2021R1A2C1091301), the framework of international cooperation program managed by the National Research Foundation of Korea (No. 2021K2A9A2A06037540), Korean Fund for Regenerative Medicine funded by Ministry of Science and ICT, and Ministry of Health and Welfare (No. 2021M3E5E5096420, Republic of Korea), Korea Medical Device Development Fund grant funded by the Korea government (the Ministry of Science and ICT, the Ministry of Trade, Industry and Energy, the Ministry of Health & Welfare, Republic of Korea, the Ministry of Food and Drug Safety) (Project Number: 202011B29), and the GRRC program of the Gyeo nggi Province (Grant Number GRRC-KPU2021-A01, Multi-material Machining Innovative Technology Research Center).
Author information
Authors and Affiliations
Contributions
HL contributed to conceptualization, investigation, validation, methodology, and writing—original draft. MKL contributed to investigation, validation, and methodology. GH contributed to validation and visualization. HEK contributed to project administration and supervision. JS and YN contributed to conceptualization and methodology. SO and CBY contributed to writing—revised draft. TSJ contributed to conceptualization, methodology, and writing—review and editing. HDJ contributed to conceptualization, methodology, writing—original draft, and writing—review and editing.
Corresponding authors
Ethics declarations
Conflict of interest
The authors declare that they have no known competing financial interests or personal relationships.
Ethical approval
This article does not contain any studies with human or animal subjects performed by any of the authors.
Rights and permissions
About this article
Cite this article
Lee, H., Lee, MK., Han, G. et al. Customizable design of multiple-biomolecule delivery platform for enhanced osteogenic responses via ‘tailored assembly system’. Bio-des. Manuf. 5, 451–464 (2022). https://doi.org/10.1007/s42242-022-00190-7
Received:
Accepted:
Published:
Issue Date:
DOI: https://doi.org/10.1007/s42242-022-00190-7