Abstract
Introduction
In this research, the hemodynamic performance of a 23-mm On-X bileaflet mechanical heart valve (BMHV) was investigated with the realistic geometry model of the valve and the deformable aorta in accelerating systole. In addition, the effect of ascending aorta flexibility and aortic annulus calcification on the complex blood flow characteristics were investigated.
Methods
The geometry of the aorta is derived from the medical images, and the Ogden model has been utilized for the mechanical behavior of the ascending aorta. The 3D numerical simulation by a two-way Fluid-Structure Interaction (FSI) analysis using the Arbitrary Lagrangian–Eulerian (ALE) method was performed throughout the accelerating systolic phase.
Results
The dynamics of the leaflets are investigated, and blood flow characteristics such as velocities, vorticities as well as viscous and turbulent shear stress were precisely captured in the flow domain specifically in the hinge region. Streamline results are in accordance with the previously reported data, which show that the flared On-X valves inlet yields a more uniform flow in accelerating systole. Simulations show that aorta flexibility or valve annulus calcification causes variations up to 7% in maximum fluid velocity and 20% in Turbulence Kinetic Energy (TKE).
Conclusions
In this study, the complex flow field characteristics in the new generation of BMHVs considering aorta flexibility with healthy and calcified annulus were investigated. It was found that the blood flow around the hinges region is in the danger of hemolysis and platelet activation and subsequently thromboembolism. Furthermore, the results show that similar to vessel wall deformation, considering the probable annulus calcification after valve replacement is also essential.























Similar content being viewed by others
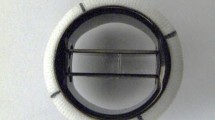
References
Akutsu, T., and D. Higuchi. Effect of the mechanical prosthetic mono-and bileaflet heart valve orientation on the flow field inside the simulated ventricle. J. Artif. Organs 3(2):126–135, 2000.
Akutsu, T., and A. Matsumoto. Influence of three mechanical bileaflet prosthetic valve designs on the three-dimensional flow field inside a simulated aorta. J. Artif. Organs 13(4):207–217, 2010.
Amindari, A., L. Saltik, K. Kirkkopru, M. Yacoub, and H. C. Yalcin. Assessment of calcified aortic valve leaflet deformations and blood flow dynamics using fluid-structure interaction modeling. Inform. Med. Unlocked 9:191–199, 2017.
ANSYS, A. ANSYS Fluent User’s Guide, 17.2. Canonsburg: ANSYS, 2016.
Bang, J. S., S. M. Yoo, and C. N. Kim. Characteristics of pulsatile blood flow through the curved bileaflet mechanical heart valve installed in two different types of blood vessels: velocity and pressure of blood flow. ASAIO J. 52(3):234–242, 2006.
Beckmann, A., A. K. Funkat, J. Lewandowski, M. Frie, M. Ernst, K. Hekmat, W. Schiller, J. F. Gummert, and W. Harringer. German heart surgery report 2016: the annual updated registry of the German Society for Thoracic and Cardiovascular Surgery. Thorac. Cardiovasc. Surg. 65(07):505–518, 2017.
Bluestein, D., E. Rambod, and M. Gharib. Vortex shedding as a mechanism for free emboli formation in mechanical heart valves. J. Biomech. Eng. 122(2):125–134, 2000.
Bonfanti, M., S. Balabani, M. Alimohammadi, O. Agu, S. Homer-Vanniasinkam, and V. Díaz-Zuccarini. A simplified method to account for wall motion in patient-specific blood flow simulations of aortic dissection: Comparison with fluid-structure interaction. Med. Eng. Phys. 58:72–79, 2018.
Cheng, R., Y. G. Lai, and K. B. Chandran. Three-dimensional fluid-structure interaction simulation of bileaflet mechanical heart valve flow dynamics. Ann. Biomed. Eng. 32(11):1471–1483, 2004.
Chien, H. L., B. W. Huang, and J. H. Kuang. The Ogden model for coronary artery mechanical behaviors. Life Sci. J. 8(4):430–437, 2011.
Dasi, L. P., L. Ge, H. A. Simon, F. Sotiropoulos, and A. P. Yoganathan. Vorticity dynamics of a bileaflet mechanical heart valve in an axisymmetric aorta. Phys. Fluids 19(6):067105, 2007.
De Tullio, M. D., A. Cristallo, E. Balaras, and R. Verzicco. Direct numerical simulation of the pulsatile flow through an aortic bileaflet mechanical heart valve. J. Fluid Mech. 622:259–290, 2009.
Dumont, K., J. M. A. Stijnen, J. Vierendeels, F. N. Van De Vosse, and P. R. Verdonck. Validation of a fluid-structure interaction model of a heart valve using the dynamic Karimi method in fluent. Comput Methods Biomech. Biomed. Eng. 7(3):139–146, 2004.
Dumont, K., J. Vierendeels, R. Kaminsky, G. Van Nooten, P. Verdonck, and D. Bluestein. Comparison of the hemodynamic and thrombogenic performance of two bileaflet mechanical heart valves using a CFD/FSI model. J. Biomech. Eng. 129(4):558–565, 2007.
Evangelista, A., F. A. Flachskampf, R. Erbel, F. Antonini-Canterin, C. Vlachopoulos, G. Rocchi, R. Sicari, P. Nihoyannopoulos, J. Zamorano, Document Reviewers, and M. Pepi. Echocardiography in aortic diseases: EAE recommendations for clinical practice. Eur. J. Echocardiogr. 11(8):645–658, 2010.
Ge, L., L. P. Dasi, F. Sotiropoulos, and A. P. Yoganathan. Characterization of hemodynamic forces induced by mechanical heart valves: Reynolds vs. viscous stresses. Ann. Biomed. Eng. 36(2):276–297, 2008.
Ge, L., H. L. Leo, F. Sotiropoulos, and A. P. Yoganathan. Flow in a mechanical bileaflet heart valve at laminar and near-peak systole flow rates: CFD simulations and experiments. J. Biomech. Eng. 127(5):782–797, 2005.
Govindarajan, V., H. S. Udaykumar, and K. B. Chandran. Two-dimensional simulation of flow and platelet dynamics in the hinge region of a mechanical heart valve. J. Biomech. Eng. 131(3):031002, 2009.
Hanafizadeh, P., N. Mirkhani, M. R. Davoudi, M. Masouminia, and K. Sadeghy. Non-Newtonian blood flow simulation of diastolic phase in bileaflet mechanical heart valve implanted in a realistic aortic root containing coronary arteries. Artif. Organs 40(10):E179–E191, 2016.
Hedayat, M., H. Asgharzadeh, and I. Borazjani. Platelet activation of mechanical versus bioprosthetic heart valves during systole. J. Biomech. 56:111–116, 2017.
Johansen, P. Mechanical heart valve cavitation. Expert Rev. Med. Devices 1(1):95–104, 2004.
Karimi, A., M. Navidbakhsh, M. Alizadeh, and A. Shojaei. A comparative study on the mechanical properties of the umbilical vein and umbilical artery under uniaxial loading. Artery Res. 8(2):51–56, 2014.
King, M. J., J. Corden, T. David, and J. Fisher. A three-dimensional, time-dependent analysis of flow through a bileaflet mechanical heart valve: comparison of experimental and numerical results. J. Biomech. 29(5):609–618, 1996.
King, M. J., T. David, and J. Fisher. An initial parametric study on fluid flow through bileaflet mechanical heart valves using computational fluid dynamics. Proc. Inst. Mech. Eng. 208(2):63–72, 1994.
Kuan, Y. H., F. Kabinejadian, V. T. Nguyen, B. Su, A. P. Yoganathan, and H. L. Leo. Comparison of hinge microflow fields of bileaflet mechanical heart valves implanted in different sinus shape and downstream geometry. Comput. Methods biomech. Biomed. Eng. 18(16):1785–1796, 2015.
Kwon, Y. J. Numerical analysis for the structural strength comparison of St. Jude Medical and Edwards MIRA bileaflet mechanical heart valve prostheses. J. Mech. Sci. Technol. 24(2):461–469, 2010.
Lee, H., A. Homma, and Y. Taenaka. Hydrodynamic characteristics of bileaflet mechanical heart valves in an artificial heart: cavitation and closing velocity. Artif. Organs 31(7):532–537, 2007.
Li, C. P., and P. C. Lu. Numerical comparison of the closing dynamics of a new trileaflet and a bileaflet mechanical aortic heart valve. J. Artif. Organs 15(4):364–374, 2012.
Mao, S. S., N. Ahmadi, B. Shah, D. Beckmann, A. Chen, L. Ngo, F. R. Flores, Y. lin Gao, and M. J. Budoff. Normal thoracic aorta diameter on cardiac computed tomography in healthy asymptomatic adults: impact of age and gender. Acad. Radiol. 15(7):827–834, 2008.
Mirkhani, N., M. R. Davoudi, P. Hanafizadeh, D. Javidi, and N. Saffarian. On-X heart valve prosthesis: numerical simulation of hemodynamic performance in accelerating systole. Cardiovasc. Eng. Technol. 7(3):223–237, 2016.
Morbiducci, U., R. Ponzini, M. Nobili, D. Massai, F. M. Montevecchi, D. Bluestein, and A. Redaelli. Blood damage safety of prosthetic heart valves. Shear-induced platelet activation and local flow dynamics: a fluid-structure interaction approach. J. Biomech. 42(12):1952–1960, 2009.
Nichols, W. W. Vascular impedance. McDonald’s blood flow in arteries: theoretical, experimental and clinical principles. Baco Raton: CRC Press, pp. 243–283, 1998.
Nobili, M. A. T. T. E. O., G. Passoni, and A. Redaelli. Two fluid-structure approaches for 3D simulation of St. Jude Medical bileaflet valve opening. J. Appl. Biomater. Biomech. 5(1):49–59, 2007.
Nowak, M., B. Melka, M. Rojczyk, M. Gracka, A. J. Nowak, A. Golda, W. P. Adamczyk, B. Isaac, R. A. Białecki, and Z. Ostrowski. The protocol for using elastic wall model in modeling blood flow within human artery. Eur. J. Mech. B 77:273–280, 2019.
On-X Prosthetic Heart Valve Design and Features - On-X Life Technologies, Inc. https://www.onxlti.com/medical-professionals/on-x-prosthetic-heart-valve-design-and-features.
Palatianos, G. M., A. M. Laczkovics, P. Simon, J. L. Pomar, D. E. Birnbaum, H. H. Greve, and A. Haverich. Multicentered European study on safety and effectiveness of the On-X prosthetic heart valve: intermediate follow-up. Ann. Thorac. Surg. 83(1):40–46, 2007.
Siu, S. C., and C. K. Silversides. Bicuspid aortic valve disease. J. Am. Coll. Cardiol. 55(25):2789–2800, 2010.
Sotiropoulos, F., and I. Borazjani. A review of state-of-the-art numerical methods for simulating flow through mechanical heart valves. Med. Biol. Eng. Comput. 47(3):245–256, 2009.
Sturla, F., M. Ronzoni, M. Vitali, A. Dimasi, R. Vismara, G. Preston-Maher, G. Burriesci, E. Votta, and A. Redaelli. Impact of different aortic valve calcification patterns on the outcome of transcatheter aortic valve implantation: a finite element study. J. Biomech. 49(12):2520–2530, 2016.
Yeh, H. H., D. Grecov, and S. Karri. Computational modelling of bileaflet mechanical valves using fluid-structure interaction approach. J. Med. Biol. Eng. 34(5):482–486, 2014.
Yun, B. M., J. Wu, H. A. Simon, S. Arjunon, F. Sotiropoulos, C. K. Aidun, and A. P. Yoganathan. A numerical investigation of blood damage in the hinge area of aortic bileaflet mechanical heart valves during the leakage phase. Ann. Biomed. Eng. 40(7):1468–1485, 2012.
Zakerzadeh, R., M. C. Hsu, and M. S. Sacks. Computational methods for the aortic heart valve and its replacements. Expert Rev. Med. Devices 14(11):849–866, 2017.
Author information
Authors and Affiliations
Corresponding author
Additional information
Associate Editors Francesco Migliavacca, Ajit Yoganathan oversaw the review of this article.
Publisher's Note
Springer Nature remains neutral with regard to jurisdictional claims in published maps and institutional affiliations.
Rights and permissions
About this article
Cite this article
Sadipour, M., Hanafizadeh, P., Sadeghy, K. et al. Effect of Aortic Wall Deformation with Healthy and Calcified Annulus on Hemodynamic Performance of Implanted On-X Valve. Cardiovasc Eng Tech 11, 141–161 (2020). https://doi.org/10.1007/s13239-019-00453-y
Received:
Accepted:
Published:
Issue Date:
DOI: https://doi.org/10.1007/s13239-019-00453-y