Abstract
The development of bimetallic sites on supported catalysts has enhanced catalytic performance compared to the corresponding monometallic sites. To determine the impact of TiO2 addition on the development of the active phase on CoMo/Al2O3 catalyst in the hydrogenation of Pyrolysis Gasoline (PyGas), a range of CoMo catalysts supported on different Al2O3 supports were created. The relevant operating parameters were utilized in a fixed bed reactor to assess how the TiO2 loading component affected the activity of such systems: T = 270–390 °C, P = 25 bar, WHSV = 4 h−1, H2: HC molar ratio = 10. The catalytic activity and selectivity of the catalysts were connected to their physical characteristics, which were examined using various characterization methods (N2 adsorption–desorption isotherms, FESEM, XRD, TPR, NH3-TPD, and FTIR spectroscopy). The XRD outcomes indicate that the Ti-containing supported catalysts appeared as anatase TiO2 species. TiO2 may alter the support and active metal interaction, enhancing molybdenum’s reducibility. The alteration of the electrical characteristics of tetrahedral and octahedral molybdenum species by titania seems to have been discovered to improve the surface acidity while simultaneously enhancing their reductive behavior. Moreover, the CoMo/Al2O3–TiO2 with a titania concentration of 5 wt% may effectively eliminate over 96% of Styrene molecules in PyGas.
Graphical Abstract








Similar content being viewed by others
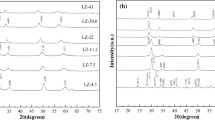
References
Gaspar AB, dos Santos GR, de Souza Costa R, da Silva MAP (2008) Hydrogenation of synthetic PYGAS—effects of zirconia on Pd/Al2O3. Catal Today 133:400–405
Esmaeili-Faraj AH et al (2021) Design of a neuro-based computing paradigm for simulation of industrial olefin plants. Chem Eng Technol 44:1382–1389
Reddy KM, Pokhriyal SK, Ratnasamy P, Sivasanker S (1992) Reforming of pyrolysis gasoline over platinum-alumina catalysts containing MFI type zeolites. Appl Catal A 83:1–13
Zhu J et al (2012) Synthesis of Ni–Mo and Co–Mo–Ni nano-sulfides and their stable catalysis on complicated full-ranged pyrolysis gasoline hydrorefinery. RSC Adv 2:8957–8961
Cheng Y-M, Chang J-R, Wu J-C (1986) Kinetic study of pyrolysis gasoline hydrogenation over supported palladium catalyst. Appl Catal 24:273–285
Metaxas KC, Papayannakos NG (2008) Studying the internal mass transfer phenomena inside a Ni/Al2O3 catalyst for benzene hydrogenation. Chem Eng J 140:352–357
Savva PG et al (2008) Benzene hydrogenation over Ni/Al2O3 catalysts prepared by conventional and sol–gel techniques. Appl Catal B 79:199–207
Betti CP et al (2021) Activity and sulfur resistance of co-impregnated bimetallic PdNi/γ-Al2O3 catalysts during hydrogenation of styrene. Braz J Chem Eng 38:315–326
Nijhuis TA, Dautzenberg FM, Moulijn JA (2003) Modeling of monolithic and trickle-bed reactors for the hydrogenation of styrene. Chem Eng Sci 8(7):1113–1124
Zhou ZM et al (2007) Kinetics of the selective hydrogenation of pyrolysis gasoline. Chem Eng Technol Ind Chem Equip Process Eng 30:105–111
Enache DI, Landon P, Lok CM, Pollington SD, Stitt EH (2005) Direct comparison of a trickle bed and a monolith for hydrogenation of pyrolysis gasoline. Ind Eng Chem Res 44:9431–9439
Hoffer BW et al (2000) Stability of highly dispersed Ni/Al2O3 catalysts: effects of pretreatment. J Catal 192:432–440
Hoffer BW et al (2004) Enhancing the start-up of pyrolysis gasoline hydrogenation reactors by applying tailored ex situ presulfided Ni/Al2O3 catalysts. Fuel 83:1–8
Duan A et al (2007) Characterization and activity of Mo supported catalysts for diesel deep hydrodesulphurization. Catal Today 119:13–18
Barrera MC et al (2004) Highly active MoS2 on wide-pore ZrO2–TiO2 mixed oxides. Catal Today 98:131–139
Damyanova S, Petrov L, Centeno MA, Grange P (2002) Characterization of molybdenum hydrodesulfurization catalysts supported on ZrO2-Al2O3 and ZrO2-SiO2 carriers. Appl Catal A 224:271–284
Esmaeili-Faraj SH, Nasr EM (2017) Influence of SiO2 and graphene oxide nanoparticles on efficiency of biological removal process. Environ Technol 38(21):2763–2774
Mendoza-Serna R et al (2003) Preparation and characterization of porous SiO2-Al2O3-ZrO2 prepared by the sol-gel process. J Porous Mater 10:31–39
Duan A et al (2009) Hydrodesulphurization performance of NiW/TiO2-Al2O3 catalyst for ultra clean diesel. Catal Today 140:187–191
Huang W et al (2008) Ti-modified alumina supports prepared by sol–gel method used for deep HDS catalysts. Catal Today 131:314–321
Ramírez J, Rayo P, Gutiérrez-Alejandre A, Ancheyta J, Rana MS (2005) Analysis of the hydrotreatment of Maya heavy crude with NiMo catalysts supported on TiO2-Al2O3 binary oxides: effect of the incorporation method of Ti. Catal Today 109:54–60
Wei ZB et al (1998) Hydrodesulfurization activity of NiMo/TiO2Al2O3 catalysts. Appl Catal A 167:39–48
Climent MJ, Corma A, Iborra S (2011) Heterogeneous catalysts for the one-pot synthesis of chemicals and fine chemicals. Chem Rev 111:1072–1133
Arena F, Cum G, Gallo R, Parmaliana A (1996) Palladium catalysts supported on oligomeric aramides in the liquid-phase hydrogenation of phenylacetylene. J Mol Catal A 110:235–242
Yasunori N et al (2005) The effect of astaxanthin on retinal capillary blood flow in normal volunteers. J Clin Ther Med 21:537–542
Jiao Z-F et al (2019) Turning the product selectivity of nitrile hydrogenation from primary to secondary amines by precise modification of Pd/SiC catalysts using NiO nanodots. Catal Sci Technol 9:2266–2272
Yu L, Liu G, Wang Z, Zhou Y, Ye H (2015) A core–shell structured Si–Al@Al2O3 as novel support of Pd catalyst. Catal Commun 68:36–40
Li Y et al (2013) A novel modification method for nickel foam support and synthesis of a metal-supported hierarchical monolithic Ni@Pd catalyst for benzene hydrogenation. Chem Eng J 226:166–170
Liu P et al (2016) Photochemical route for synthesizing atomically dispersed palladium catalysts. Science 352:797–800
Carrara N et al (2015) Selective hydrogenation by novel composite supported Pd egg-shell catalysts. Catal Commun 61:72–77
Betti C et al (2016) Kinetic study of the selective hydrogenation of styrene over a Pd egg-shell composite catalyst. React Kinet Mech Catal 117:283–306
Betti CP et al (2013) Sulfur resistance of Pt-W catalysts. J Chem. https://doi.org/10.1155/2013/502014
Betti C et al (2018) More active and sulfur resistant bimetallic Pd-Ni catalysts. Quim Nova 41:151–156
Breysse M, Afanasiev P, Geantet C, Vrinat M (2003) Overview of support effects in hydrotreating catalysts. Catal Today 86:5–16
Topsoe H, Clausen BS, Massoth FE (1996) Hydrotreating catalysis science and technology. Springer-Verlag, New York
Popov A et al (2010) Bio-oils hydrodeoxygenation: adsorption of phenolic molecules on oxidic catalyst supports. J Phys Chem C 114:15661–15670
Centeno A, Laurent E, Delmon B (1995) Influence of the support of CoMo sulfide catalysts and of the addition of potassium and platinum on the catalytic performances for the hydrodeoxygenation of carbonyl, carboxyl, and guaiacol-type molecules. J Catal 154:288–298
Yang Y, Gilbert A, Xu CC (2009) Hydrodeoxygenation of bio-crude in supercritical hexane with sulfided CoMo and CoMoP catalysts supported on MgO: a model compound study using phenol. Appl Catal A 360:242–249
Laurenti D, Afanasiev P, Geantet C (2011) Hydrodeoxygenation of guaiacol with CoMo catalysts. Part I: promoting effect of cobalt on HDO selectivity and activity. Appl Catal B 101:239–245
Bu Q et al (2012) A review of catalytic hydrodeoxygenation of lignin-derived phenols from biomass pyrolysis. Bioresour Technol 124:470–477
Esmaeili-Faraj SH et al (2021) Diesel fuel desulfurization by alumina/polymer nanocomposite membrane: experimental analysis and modeling by the response surface methodology. Chem Eng Process Process Intensif 164:108396
Ramírez J et al (2004) The role of titania in supported Mo, CoMo, NiMo, and NiW hydrodesulfurization catalysts: analysis of past and new evidences. Catal Today 98:19–30
Chen N, Gong S, Qian EW (2015) Effect of reduction temperature of NiMoO3-x/SAPO-11 on its catalytic activity in hydrodeoxygenation of methyl laurate. Appl Catal B 174:253–263
Nguyen TT, Shinozaki A, Qian EW (2017) Hydrodesulfurization, hydrodenitrogenation and hydrodearomatization over CoMo/SAPO-11-Al2O3 catalysts. J Jpn Pet Inst 60:301–310
Nguyen TT, Imai K, Pu J, Qian EW (2018) Effect of TiO2 coating on morphology of active phase on sulfided CoMo/Al2O3 hydrotreating catalysts. Energy Fuels 32:1665–1673
Keivanimehr F, Habibzadeh S, Mokhtarian M (2022) Enhanced product quality through hydrodesulfurization of pyrolysis gasoline over a mixed metal oxide catalyst: an experimental and DFT study. Fuel 317:123458
Zhu H et al (2005) Dispersion behaviors of molybdena on titania (rutile and/or anatase). J Phys Chem B 109:11720–11726
Modabberasl A, Pirhoushyaran T, Esmaeili-Faraj SH (2022) Synthesis of CoFe2O4 magnetic nanoparticles for application in photocatalytic removal of azithromycin from wastewater. Sci Rep 12(1):19171
Mouret G, Mozet K, Muhr H, Plasari E, Martin M (2007) Role of the precipitation device on the properties of Al2O3-TiO2 mixed oxides. In: Proceedings of European Congress of Chemical Engineering (ECCE-6), Copenhagen, 16–20 September 2007
Xu J et al (2008) Synthesis and photoluminescence of well-dispersible anatase TiO2 nanoparticles. J Colloid Interface Sci 318:29–34
Tiwari R, Rana BS, Kumar R, Sinha AK (2012) TiO2-ZrO2 binary oxides for effective hydrodesulfurization catalysts. Open Catal J 5:39–49
Choudhury B, Choudhury A (2012) Luminescence characteristics of cobalt doped TiO2 nanoparticles. J Lumin 132:178–184
Bhattacharyya K et al (2014) Effect of Mo-incorporation in the TiO2 lattice: a mechanistic basis for photocatalytic dye degradation. J Phys Chem C 118:15946–15962
Majeed J et al (2015) Correlation of Mo dopant and photocatalytic properties of Mo incorporated TiO2: an EXAFS and photocatalytic study. RSC Adv 5:90932–90940
Escobar J, Antonio De Los Reyes J, Viveros T (2003) Nickel on TiO2-modified Al2O3 sol–gel oxides: effect of synthesis parameters on the supported phase properties. Appl Catal A 253:151–163
Tavizón-Pozos JA, Suárez-Toriello VA, del los Ángel P, de Reyes JA (2016) Hydrodeoxygenation of phenol over sulfided CoMo catalysts supported on a mixed Al2O3-TiO2 oxide. Int J Chem React Eng 14:1211–1223
Subramanian V, Choi J, Seebauer EG, Masel RI (2007) TiO2–Al2O3 as a support for propane partial oxidation over Rh. Catal Lett 113:13–18
Yuan P, Cui C, Han W, Bao X (2016) The preparation of Mo/γ-Al2O3 catalysts with controllable size and morphology via adjusting the metal-support interaction and their hydrodesulfurization performance. Appl Catal A 524:115–125
Ma X et al (2015) Alumina supported molybdenum catalyst for lignin valorization: effect of reduction temperature. Bioresour Technol 192:17–22
Shah S, Marin-Flores OG, Chinnathambi K, Norton MG, Ha S (2016) Partial oxidation of surrogate Jet-A fuel over SiO2 supported MoO2. Appl Catal B 193:133–140
Ji J et al (2014) Towards an efficient CoMo/γ-Al2O3 catalyst using metal amine metallate as an active phase precursor: enhanced hydrogen production by ammonia decomposition. Int J Hydrogen Energy 39:12490–12498
Yamada M, Koizumi N, Yamazaki M (1999) High pressure (≤ 5.1 MPa) DRIFT study on surface structure of Co–Mo/Al2O3 and Ni–Mo/Al2O3 using NO as probe molecule. Catal Today 50:3–8
Damyanova S, Spojakina A, Jiratova K (1995) Effect of mixed titania-alumina supports on the phase composition of NiMo/TiO2Al2O3 catalysts. Appl Catal A 125:257–269
Wei Z-B, **n Q, Guo XX, Grange P, Delmon B (1991) Titania-modified hydrodesulfurization catalysts. 2. Dispersion state and catalytic activity of molybdena supported on titania alumina carrier. Appl Catal A 75:179
Zhang B et al (2016) Synthesis of Ni/mesoporous ZSM-5 for direct catalytic conversion of cellulose to hexitols: modulating the pore structure and acidic sites via a nanocrystalline cellulose template. Green Chem 18:3315–3323
Miyamoto T, Katada N, Kim J-H, Niwa M (1998) Acidic property of MFI-type gallosilicate determined by temperature-programmed desorption of ammonia. J Phys Chem B 102:6738–6745
Toyoda T, Minami T, Qian EW (2013) Mixed alcohol synthesis over sulfided molybdenum-based catalysts. Energy Fuels 27:3769–3777
Acknowledgements
This research was supported by Nouri Petrochemical Company and we sincerely thank our colleagues from Research and Development Department who provided insight and expertise that greatly assisted the research.
Author information
Authors and Affiliations
Corresponding author
Ethics declarations
Conflict of interest
On behalf of all authors, I (Mehdi Ardjmand, corresponding author) state that there is no conflict of interest.
Additional information
Publisher's Note
Springer Nature remains neutral with regard to jurisdictional claims in published maps and institutional affiliations.
Rights and permissions
Springer Nature or its licensor (e.g. a society or other partner) holds exclusive rights to this article under a publishing agreement with the author(s) or other rightsholder(s); author self-archiving of the accepted manuscript version of this article is solely governed by the terms of such publishing agreement and applicable law.
About this article
Cite this article
Parvizi, M.R., Ardjmand, M. & Habibzadeh, S. Elucidation of the Effect of TiO2 in the Synthesized Nanocatalyst of Co–Mo@Al2O3–TiO2 for the Hydrogenation of C6–C8 Olefins. Catal Lett 154, 2328–2339 (2024). https://doi.org/10.1007/s10562-023-04475-z
Received:
Accepted:
Published:
Issue Date:
DOI: https://doi.org/10.1007/s10562-023-04475-z